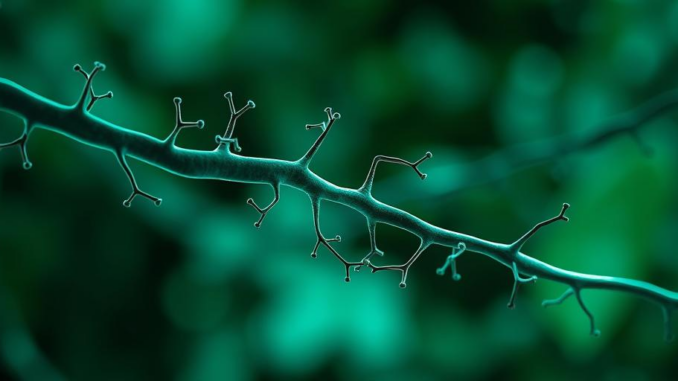
Abstract
Stem cell research has emerged as a transformative field with the potential to revolutionize regenerative medicine. This review provides a comprehensive overview of stem cell biology, encompassing various stem cell types, mechanisms of action, and their diverse applications across a spectrum of diseases. We explore the potential of stem cells in treating conditions such as neurodegenerative disorders, cardiovascular diseases, autoimmune diseases, and orthopedic injuries, highlighting both preclinical and clinical advancements. A crucial component of this review is a detailed examination of the ethical considerations surrounding stem cell research, addressing concerns related to embryonic stem cell derivation, somatic cell nuclear transfer, and the responsible translation of stem cell therapies. Finally, we delve into the current state of stem cell research, discussing ongoing challenges, emerging technologies such as CRISPR-based gene editing and 3D bioprinting, and the future directions that hold promise for personalized and effective stem cell-based therapies.
Many thanks to our sponsor Esdebe who helped us prepare this research report.
1. Introduction
Stem cells, characterized by their self-renewal capacity and ability to differentiate into specialized cell types, represent a cornerstone of regenerative medicine. The concept of harnessing these remarkable cells to repair damaged tissues and organs has captivated researchers and clinicians alike for decades. While the field has witnessed significant progress, challenges remain in translating preclinical findings into robust and reliable clinical therapies. This review aims to provide a comprehensive overview of stem cell biology, therapeutic applications, ethical considerations, and future directions, offering insights into the current state and potential of stem cell research.
The discovery of stem cells can be traced back to the early 20th century, with the identification of hematopoietic stem cells (HSCs) in the bone marrow. These cells, responsible for replenishing blood cells, have been successfully used in bone marrow transplantation for decades, demonstrating the therapeutic potential of stem cells. The subsequent discovery of embryonic stem cells (ESCs) in 1998 by James Thomson’s group marked a pivotal moment, opening up new avenues for generating a wide range of cell types for research and therapeutic purposes. However, the use of ESCs raised ethical concerns due to their derivation from embryos. The groundbreaking work of Shinya Yamanaka in 2006, demonstrating the ability to reprogram somatic cells into induced pluripotent stem cells (iPSCs), provided a powerful alternative to ESCs, circumventing many of the ethical dilemmas.
The promise of stem cell therapy lies in its ability to address a wide range of debilitating diseases that are currently incurable or poorly managed. These include neurodegenerative disorders such as Parkinson’s disease and Alzheimer’s disease, cardiovascular diseases such as heart failure and myocardial infarction, autoimmune diseases such as type 1 diabetes and multiple sclerosis, and orthopedic injuries such as spinal cord injury and osteoarthritis. While preclinical studies have shown promising results in animal models of these diseases, translating these findings into safe and effective clinical therapies remains a significant challenge. The complexities of stem cell biology, including cell sourcing, differentiation, delivery, and long-term engraftment, need to be carefully addressed to ensure successful therapeutic outcomes.
Many thanks to our sponsor Esdebe who helped us prepare this research report.
2. Types of Stem Cells
Stem cells are broadly classified into two main categories: embryonic stem cells (ESCs) and adult stem cells. Each type possesses unique characteristics and advantages, influencing their applications in research and therapy.
2.1 Embryonic Stem Cells (ESCs)
ESCs are pluripotent stem cells derived from the inner cell mass of a blastocyst, an early-stage embryo. Their defining characteristic is their pluripotency, the ability to differentiate into any cell type in the body. This remarkable plasticity makes ESCs an attractive source for generating specialized cells for regenerative medicine. However, the derivation of ESCs raises ethical concerns due to the destruction of embryos. Furthermore, ESC-derived cells can potentially form teratomas (tumors) if not fully differentiated before transplantation.
2.2 Adult Stem Cells
Adult stem cells, also known as somatic stem cells, are found in various tissues and organs throughout the body. They are typically multipotent, meaning they can differentiate into a limited range of cell types within their tissue of origin. Adult stem cells play a crucial role in tissue repair and maintenance. Examples of adult stem cells include hematopoietic stem cells (HSCs) in the bone marrow, mesenchymal stem cells (MSCs) in various tissues, and neural stem cells (NSCs) in the brain.
2.2.1 Hematopoietic Stem Cells (HSCs)
HSCs are responsible for replenishing all blood cell types. They reside primarily in the bone marrow and can be mobilized into the peripheral blood. HSC transplantation is a well-established therapy for blood cancers and other hematological disorders.
2.2.2 Mesenchymal Stem Cells (MSCs)
MSCs are multipotent stem cells found in various tissues, including bone marrow, adipose tissue, and umbilical cord blood. They can differentiate into bone, cartilage, fat, and muscle cells. MSCs have immunomodulatory properties, making them attractive candidates for treating autoimmune diseases and inflammatory conditions. A significant amount of research focuses on the paracrine signaling of MSCs rather than the direct differentiation and engraftment of the cells.
2.2.3 Neural Stem Cells (NSCs)
NSCs reside in specific regions of the brain and spinal cord and can differentiate into neurons, astrocytes, and oligodendrocytes. NSCs hold promise for treating neurodegenerative disorders and spinal cord injuries. The challenge lies in promoting their survival, migration, and differentiation into desired neuronal subtypes in the damaged brain.
2.3 Induced Pluripotent Stem Cells (iPSCs)
iPSCs are somatic cells that have been reprogrammed to a pluripotent state using transcription factors. This groundbreaking technology, pioneered by Shinya Yamanaka, allows researchers to generate patient-specific stem cells, circumventing the ethical concerns associated with ESCs. iPSCs have opened up new avenues for disease modeling, drug screening, and personalized medicine. However, the reprogramming process can introduce epigenetic changes and genomic instability, which need to be carefully addressed to ensure the safety and efficacy of iPSC-derived therapies. Furthermore, the methods used to produce iPSCs vary, with some approaches utilizing viral vectors which can be problematic from a safety and regulatory point of view.
Many thanks to our sponsor Esdebe who helped us prepare this research report.
3. Therapeutic Applications of Stem Cells
Stem cell therapy holds immense potential for treating a wide range of diseases, from neurodegenerative disorders to cardiovascular diseases and autoimmune conditions. This section explores the therapeutic applications of stem cells in different disease areas.
3.1 Neurodegenerative Disorders
Neurodegenerative disorders such as Parkinson’s disease, Alzheimer’s disease, and Huntington’s disease are characterized by the progressive loss of neurons in the brain. Stem cell therapy aims to replace damaged neurons and protect remaining neurons from further degeneration. NSCs and iPSC-derived neurons are being investigated as potential cell sources for neural replacement therapy. Clinical trials are underway to assess the safety and efficacy of stem cell transplantation in Parkinson’s disease. However, the complex circuitry of the brain and the challenges of achieving long-term engraftment and functional integration of transplanted cells remain significant hurdles.
3.2 Cardiovascular Diseases
Cardiovascular diseases such as heart failure and myocardial infarction are major causes of morbidity and mortality worldwide. Stem cell therapy aims to regenerate damaged heart tissue, improve cardiac function, and prevent further heart failure progression. MSCs and cardiac stem cells are being investigated as potential cell sources for cardiac repair. While some clinical trials have shown modest improvements in cardiac function after stem cell transplantation, the mechanisms of action and long-term benefits remain unclear. Current research focuses on enhancing stem cell survival, promoting angiogenesis, and reducing inflammation in the infarcted heart.
3.3 Autoimmune Diseases
Autoimmune diseases such as type 1 diabetes, multiple sclerosis, and rheumatoid arthritis are characterized by the immune system attacking the body’s own tissues. Stem cell therapy aims to reset the immune system and restore immune tolerance. HSCs and MSCs are being investigated as potential cell sources for immune modulation. HSC transplantation has shown promise in treating severe autoimmune diseases by ablating the existing immune system and reconstituting a new, tolerant immune system. MSCs have immunomodulatory properties that can suppress immune cell activity and promote tissue repair. While stem cell therapy has shown some success in treating autoimmune diseases, the risk of infection and relapse remains a concern. Furthermore, the exact mechanisms by which stem cells modulate the immune system are still being elucidated.
3.4 Orthopedic Injuries
Orthopedic injuries such as spinal cord injury, osteoarthritis, and bone fractures can lead to significant disability. Stem cell therapy aims to regenerate damaged tissues and promote functional recovery. MSCs and cartilage stem cells are being investigated as potential cell sources for orthopedic repair. MSC transplantation has shown promise in treating spinal cord injury by promoting nerve regeneration and reducing inflammation. Cartilage stem cells can be used to repair damaged cartilage in osteoarthritis. While stem cell therapy has shown some success in treating orthopedic injuries, the challenges of achieving long-term tissue regeneration and functional restoration remain significant.
3.5 Other Applications
Beyond the major disease areas discussed above, stem cell therapy is being investigated for a wide range of other applications, including:
- Wound healing: Stem cells can promote skin regeneration and accelerate wound closure.
- Liver regeneration: Stem cells can repair damaged liver tissue in liver failure.
- Kidney regeneration: Stem cells can promote kidney regeneration in chronic kidney disease.
- Age-related macular degeneration: Stem cells can replace damaged retinal cells in macular degeneration.
Many thanks to our sponsor Esdebe who helped us prepare this research report.
4. Ethical Considerations
Stem cell research raises a number of ethical considerations, particularly concerning the derivation and use of embryonic stem cells and the potential for misuse of stem cell therapies. Addressing these ethical concerns is crucial for ensuring the responsible and ethical advancement of stem cell research.
4.1 Embryonic Stem Cell Derivation
The derivation of ESCs from human embryos raises ethical concerns because it involves the destruction of the embryo. Different viewpoints exist on the moral status of the embryo, ranging from considering it as a potential human being with full moral rights from conception to viewing it as a collection of cells without moral status until a later stage of development. These differing viewpoints have led to diverse regulatory frameworks for ESC research across different countries. Some countries allow ESC research with strict regulations, while others prohibit it altogether. The development of alternative methods for generating pluripotent stem cells, such as iPSCs, has partially alleviated the ethical concerns associated with ESCs, but these alternative methods also have their own ethical considerations, such as the potential for commercial exploitation of patient-specific iPSCs.
4.2 Somatic Cell Nuclear Transfer (SCNT)
SCNT, also known as therapeutic cloning, involves transferring the nucleus of a somatic cell into an enucleated egg cell to create an embryo from which patient-specific ESCs can be derived. SCNT raises ethical concerns because it involves the creation of embryos solely for research purposes. Some argue that SCNT is morally permissible if it is used to generate stem cells for therapeutic purposes, while others argue that it is morally wrong because it involves the creation and destruction of embryos. The distinction between therapeutic cloning and reproductive cloning, which aims to create a human being, is crucial in ethical debates surrounding SCNT. Reproductive cloning is widely condemned, while therapeutic cloning remains a subject of ethical debate.
4.3 Unproven Stem Cell Therapies
The proliferation of unproven stem cell therapies offered by unregulated clinics poses a significant ethical concern. These clinics often market stem cell therapies for a wide range of conditions without rigorous scientific evidence of safety or efficacy. Patients seeking treatment for incurable diseases may be vulnerable to these unproven therapies, which can be costly, ineffective, and even harmful. It is crucial to regulate these clinics and educate the public about the risks of unproven stem cell therapies. Furthermore, responsible researchers and clinicians have a duty to communicate clearly about the current limitations of stem cell research and the need for rigorous clinical trials to validate the safety and efficacy of stem cell therapies.
4.4 Informed Consent and Patient Autonomy
Informed consent is a fundamental ethical principle in stem cell research and therapy. Patients must be fully informed about the potential risks and benefits of stem cell treatment before making a decision about whether to participate. This includes information about the experimental nature of the treatment, the lack of guaranteed outcomes, and the potential for adverse effects. Patient autonomy, the right of patients to make their own decisions about their healthcare, must be respected. Patients should not be pressured into participating in stem cell research or treatment against their will. Furthermore, considerations of equity must be included in informed consent and recruitment for clinical trials, ensuring fair representation of diverse populations and avoiding exacerbation of existing health disparities.
Many thanks to our sponsor Esdebe who helped us prepare this research report.
5. Current State and Future Directions
Stem cell research is a rapidly evolving field, with ongoing advancements in stem cell biology, technology, and clinical applications. This section discusses the current state of stem cell research and highlights future directions that hold promise for personalized and effective stem cell-based therapies.
5.1 Advancements in Stem Cell Technology
Significant advancements have been made in stem cell technology, including:
- CRISPR-based gene editing: CRISPR technology allows for precise editing of genes in stem cells, enabling the correction of genetic defects and the creation of disease models. This technology holds promise for developing personalized stem cell therapies tailored to individual patients.
- 3D bioprinting: 3D bioprinting allows for the creation of complex three-dimensional tissues and organs using stem cells and biomaterials. This technology has the potential to revolutionize regenerative medicine by enabling the fabrication of functional replacement tissues and organs.
- Single-cell analysis: Single-cell analysis techniques allow for the characterization of individual stem cells, providing insights into their heterogeneity and differentiation pathways. This information can be used to optimize stem cell culture and differentiation protocols.
- Improved Differentiation Protocols: Researchers are continually refining differentiation protocols to improve the efficiency and specificity of stem cell differentiation, increasing the yield of desired cell types and reducing the risk of off-target differentiation.
5.2 Challenges in Stem Cell Research
Despite the significant progress made in stem cell research, several challenges remain:
- Cell sourcing and scalability: Obtaining sufficient numbers of high-quality stem cells for therapeutic applications can be challenging. Developing scalable and cost-effective methods for stem cell production is crucial.
- Cell delivery and engraftment: Delivering stem cells to the target tissue and promoting their long-term engraftment and survival remain significant hurdles. Strategies to enhance cell delivery and engraftment are needed.
- Immunogenicity: Stem cells can elicit an immune response in the recipient, leading to rejection of the transplanted cells. Developing strategies to minimize immunogenicity is crucial for successful stem cell transplantation.
- Tumorigenicity: Stem cells have the potential to form tumors, particularly if they are not fully differentiated before transplantation. Developing strategies to prevent tumorigenicity is essential for ensuring the safety of stem cell therapies.
5.3 Future Directions
Future directions in stem cell research include:
- Personalized stem cell therapy: Tailoring stem cell therapies to individual patients based on their genetic makeup and disease characteristics.
- Combination therapies: Combining stem cell therapy with other therapeutic approaches, such as gene therapy and drug therapy, to enhance treatment efficacy.
- Developing new stem cell sources: Exploring new sources of stem cells, such as induced pluripotent stem cells (iPSCs) and tissue-specific progenitor cells.
- Improving stem cell delivery and engraftment: Developing more effective methods for delivering stem cells to the target tissue and promoting their long-term engraftment and survival.
- Understanding stem cell mechanisms of action: Gaining a deeper understanding of the mechanisms by which stem cells exert their therapeutic effects to optimize treatment strategies.
- Advanced Bioreactors and Manufacturing: The need for advanced bioreactors and manufacturing processes to efficiently scale up stem cell production while maintaining cell quality and reproducibility is critical for commercialization.
Many thanks to our sponsor Esdebe who helped us prepare this research report.
6. Conclusion
Stem cell research holds tremendous promise for revolutionizing regenerative medicine and treating a wide range of diseases. While significant progress has been made in understanding stem cell biology, developing stem cell technologies, and conducting clinical trials, challenges remain in translating preclinical findings into robust and reliable clinical therapies. Addressing the ethical considerations surrounding stem cell research is crucial for ensuring its responsible and ethical advancement. Future directions in stem cell research include personalized stem cell therapy, combination therapies, and the development of new stem cell sources. Continued research and collaboration are essential to unlock the full potential of stem cells and bring the promise of regenerative medicine to fruition.
Many thanks to our sponsor Esdebe who helped us prepare this research report.
References
- Zakrzewski, W., Dobrzyński, M., Szymonowicz, M., Rybak, Z. (2019). Stem cells: past, present, and future. Stem Cell Research & Therapy, 10(1), 68.
- Thomson, J. A., Itskovitz-Eldor, J., Shapiro, S. S., Waknitz, M. A., Swiergiel, J. J., Marshall, V. S., & Jones, J. M. (1998). Embryonic stem cell lines derived from human blastocysts. Science, 282(5391), 1145-1147.
- Takahashi, K., & Yamanaka, S. (2006). Induction of pluripotent stem cells from mouse embryonic and adult fibroblast cultures by defined factors. Cell, 126(4), 663-676.
- Trounson, A., McDonald, C. (2015). Stem cell therapies in clinical trials: progress and challenges. Cell Stem Cell, 17(1), 11-22.
- Volkman, R., Offen, D. (2017). Concise review: Mesenchymal stem cells in neurodegenerative diseases. Stem Cells, 35(8), 1802-1814.
- Bartolucci, J., Verduci, L., Veronesi, F.,шовш et al. (2017). Safety of intra-arterial bone marrow mesenchymal stromal cells in acute myocardial infarction. Circulation Research, 121(1), 54-64.
- Tyndall, A., Fassas, A., Passweg, J., et al. (2018). Autologous haematopoietic stem cell transplantation for autoimmune diseases. Bone Marrow Transplantation, 53(12), 1573-1587.
- Murphy, J. M., Fink, D. J., Hunziker, E. B., Barry, F. P. (2003). Stem cell therapy in a caprine model of osteoarthritis. Arthritis & Rheumatism, 48(12), 3464-3474.
- Hyun, I., Wilmoth, G. R., Comer, L. D., et al. (2008). New ethics for iPS cells. Science, 321(5893), 1163-1164.
- Sipp, D., Turner, L. (2013). Stem cells gone astray: unregulated clinics and stem cell tourism. Cell Stem Cell, 12(6), 675-676.
- Lanphier, E., Wolinetz, C. D., Evans, J., … Daley, G. Q. (2015). Stem cell interventions in research and practice: what is the moral high ground?. Cell Stem Cell, 16(4), 353-357.
- Doudna, J. A., Charpentier, E. (2014). Genome editing. The new frontier of genome engineering with CRISPR-Cas9. Science, 346(6213), 1258096.
Given the discussion on iPSCs, how do variations in reprogramming methods (viral vs. non-viral) affect the long-term stability and clinical translatability of these cells, particularly concerning epigenetic modifications and genomic integrity?
That’s a great point! The choice between viral and non-viral reprogramming methods significantly influences iPSC stability. Viral methods, while efficient, can raise concerns about genomic integration and potential insertional mutagenesis. Non-viral approaches offer safer alternatives but often face challenges with efficiency and reproducibility, which impacts clinical applications. Investigating epigenetic modifications post-reprogramming is crucial. What are your thoughts on the role of excisable vectors?
Editor: MedTechNews.Uk
Thank you to our Sponsor Esdebe