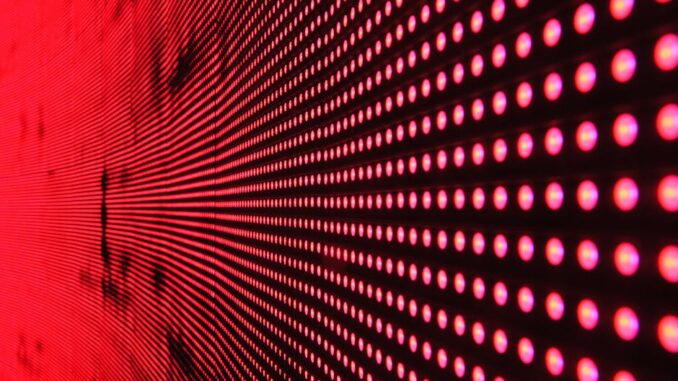
The Evolving Landscape of Genomics: Technological Advancements, Applications, Ethical Considerations, and Regulatory Challenges
Abstract
Genomics, the study of the complete set of genes and their interactions, has rapidly transformed from a nascent field to a cornerstone of biomedical research and healthcare. This report provides a comprehensive overview of the current state of genomics, focusing on recent technological advancements driving innovation, the diverse applications of genomic information in diagnostics and personalized medicine, critical ethical considerations surrounding data privacy and equity, and the increasing regulatory scrutiny faced by the genomics industry. We explore the challenges associated with data interpretation, the need for robust data infrastructure, and the evolving regulatory landscape aimed at ensuring responsible and equitable use of genomic technologies. Furthermore, we assess the overall impact of genomics on healthcare and identify key areas for future research and development.
Many thanks to our sponsor Esdebe who helped us prepare this research report.
1. Introduction
Genomics has witnessed an unprecedented surge in development and application over the past two decades. The completion of the Human Genome Project in 2003 marked a watershed moment, providing a comprehensive blueprint of human genetic information [1]. This achievement spurred the development of increasingly sophisticated and cost-effective sequencing technologies, allowing researchers and clinicians to delve deeper into the complexities of the genome. The resulting deluge of genomic data has fueled advancements in various fields, including disease diagnostics, personalized medicine, drug discovery, and agriculture. However, the rapid progress in genomics also raises significant ethical, legal, and social implications (ELSI) that demand careful consideration.
This report aims to provide a comprehensive overview of the current genomics landscape, encompassing technological advancements, applications, ethical considerations, and regulatory challenges. We will explore the ongoing innovation in sequencing technologies, data analysis methods, and genome editing techniques. We will then delve into the diverse applications of genomics in diagnostics, personalized medicine, and other areas, highlighting the potential benefits and limitations. Furthermore, we will critically examine the ethical concerns surrounding genomic data privacy, equity, and access. Finally, we will discuss the increasing regulatory scrutiny faced by the genomics industry and the need for clear and consistent guidelines to ensure responsible and ethical use of genomic technologies.
Many thanks to our sponsor Esdebe who helped us prepare this research report.
2. Technological Advancements in Genomics
The field of genomics is continuously evolving, driven by advancements in sequencing technologies, data analysis methods, and genome editing techniques. These advancements have not only reduced the cost and time required for genomic analysis but also expanded the scope of genomic research and its applications.
2.1 Sequencing Technologies
Next-generation sequencing (NGS) technologies have revolutionized genomics by enabling massively parallel sequencing of DNA and RNA. Several NGS platforms are currently available, each with its own strengths and limitations. Illumina’s sequencing-by-synthesis (SBS) technology remains the dominant platform in the market, known for its high accuracy and throughput [2]. Other platforms, such as those developed by PacBio and Oxford Nanopore, offer longer read lengths, which are particularly valuable for resolving complex genomic regions and structural variations [3].
PacBio’s Single Molecule, Real-Time (SMRT) sequencing technology allows for direct sequencing of DNA molecules without the need for amplification, providing higher accuracy and the ability to detect modified bases. Oxford Nanopore’s nanopore sequencing technology offers real-time sequencing of single DNA or RNA molecules as they pass through a protein nanopore, enabling long-read sequencing with portable and relatively inexpensive devices [4]. The development of these long-read sequencing technologies has significantly improved our ability to characterize complex genomic regions, such as repetitive sequences and structural variations, which are often difficult to analyze using short-read sequencing.
Beyond traditional NGS platforms, emerging technologies such as single-cell sequencing are enabling researchers to study the genomic profiles of individual cells, providing insights into cellular heterogeneity and the mechanisms underlying complex diseases [5]. Spatial transcriptomics, another emerging technology, allows for the mapping of gene expression patterns within tissues, providing a spatial context for understanding gene function and regulation [6].
2.2 Data Analysis Methods
The increasing volume of genomic data generated by NGS technologies has created a significant challenge for data analysis and interpretation. Sophisticated bioinformatics tools and algorithms are needed to process, analyze, and interpret these data. These tools include read alignment algorithms, variant calling algorithms, genome browsers, and pathway analysis tools.
Machine learning (ML) and artificial intelligence (AI) are playing an increasingly important role in genomic data analysis. ML algorithms can be trained to identify patterns and predict outcomes based on genomic data, enabling researchers to identify disease-causing genes, predict drug responses, and develop personalized treatment strategies [7]. AI-powered tools are also being used to automate data processing and interpretation, reducing the burden on researchers and improving the efficiency of genomic analysis.
Cloud-based platforms are becoming increasingly popular for genomic data analysis, providing researchers with access to powerful computing resources and sophisticated analytical tools [8]. These platforms also facilitate data sharing and collaboration, accelerating the pace of genomic research. The development of user-friendly interfaces and standardized data formats is making genomic data analysis more accessible to researchers with diverse backgrounds.
2.3 Genome Editing Technologies
Genome editing technologies, such as CRISPR-Cas9, have revolutionized the field of genomics by enabling precise and efficient modification of DNA sequences within living cells [9]. CRISPR-Cas9 technology utilizes a guide RNA molecule to direct the Cas9 enzyme to a specific DNA sequence, where it cleaves the DNA, allowing for the insertion, deletion, or modification of genes. This technology has a wide range of applications, including gene therapy, disease modeling, and drug discovery.
While CRISPR-Cas9 has proven to be a powerful tool, it also raises significant ethical and safety concerns. Off-target effects, where the Cas9 enzyme cleaves DNA at unintended sites, are a major concern [10]. Researchers are working to improve the specificity of CRISPR-Cas9 and develop methods for detecting and mitigating off-target effects. Other genome editing technologies, such as base editing and prime editing, offer greater precision and reduced off-target effects compared to CRISPR-Cas9 [11].
The therapeutic potential of genome editing technologies is enormous. Clinical trials are underway to evaluate the safety and efficacy of CRISPR-Cas9-based therapies for a variety of genetic diseases, including sickle cell anemia, beta-thalassemia, and cystic fibrosis [12]. However, the long-term effects of genome editing are still unknown, and careful monitoring is needed to ensure the safety of patients.
Many thanks to our sponsor Esdebe who helped us prepare this research report.
3. Applications of Genomics
Genomics has a wide range of applications in various fields, including diagnostics, personalized medicine, drug discovery, agriculture, and forensics. The ability to analyze and interpret genomic data is transforming healthcare and other industries.
3.1 Diagnostics
Genomic testing is increasingly being used for the diagnosis of genetic diseases, infectious diseases, and cancer. Genetic testing can identify individuals who are at risk for developing certain diseases, allowing for early intervention and preventive measures [13]. Diagnostic sequencing can also be used to identify the causative mutations in patients with undiagnosed genetic disorders, leading to improved diagnosis and treatment [14].
Infectious disease diagnostics is another area where genomics is making a significant impact. NGS can be used to identify pathogens, track outbreaks, and monitor the spread of antimicrobial resistance [15]. Genomic surveillance of viruses, such as SARS-CoV-2, has been crucial for understanding the evolution and transmission of the virus and for developing effective vaccines and treatments.
In cancer diagnostics, genomic testing is used to identify cancer-causing mutations, predict treatment responses, and monitor disease progression [16]. Liquid biopsies, which involve analyzing circulating tumor DNA (ctDNA) in blood samples, are becoming increasingly popular for cancer diagnosis and monitoring, offering a non-invasive alternative to traditional tissue biopsies.
3.2 Personalized Medicine
Personalized medicine, also known as precision medicine, aims to tailor medical treatment to the individual characteristics of each patient, including their genetic makeup, lifestyle, and environment [17]. Genomics plays a central role in personalized medicine by providing information about an individual’s genetic predisposition to disease and their response to different treatments.
Pharmacogenomics, the study of how genes affect a person’s response to drugs, is a key component of personalized medicine. Genomic testing can identify individuals who are likely to benefit from certain drugs or who are at risk for adverse drug reactions [18]. This information can be used to optimize drug selection and dosage, improving treatment outcomes and reducing the risk of side effects.
Personalized medicine also involves using genomic information to guide lifestyle recommendations and preventive measures. For example, individuals with a genetic predisposition to heart disease may be advised to adopt a healthy diet and exercise regularly to reduce their risk of developing the disease.
3.3 Drug Discovery
Genomics is playing an increasingly important role in drug discovery by providing insights into the genetic basis of disease and by identifying potential drug targets [19]. Genomic data can be used to identify genes that are involved in disease pathways, which can then be targeted by new drugs. Genome editing technologies, such as CRISPR-Cas9, can be used to create disease models in cells and animals, which can be used to test the efficacy of new drugs.
Genomics is also being used to identify biomarkers that can be used to predict drug responses and monitor treatment efficacy [20]. These biomarkers can be used to stratify patients into different groups based on their likelihood of responding to a particular drug, allowing for more targeted and effective treatment.
3.4 Other Applications
Genomics has numerous other applications in fields such as agriculture, forensics, and environmental science. In agriculture, genomics is being used to improve crop yields, enhance nutritional value, and develop disease-resistant crops [21]. In forensics, genomics is used for DNA fingerprinting and identifying individuals from biological samples [22]. In environmental science, genomics is used to study the diversity and function of microbial communities and to monitor environmental pollution [23].
Many thanks to our sponsor Esdebe who helped us prepare this research report.
4. Ethical Considerations
The rapid advancements in genomics raise significant ethical, legal, and social implications (ELSI) that demand careful consideration. These considerations include data privacy, equity, access, and the potential for genetic discrimination.
4.1 Data Privacy
Genomic data is highly sensitive and personal information that can reveal a great deal about an individual’s health, ancestry, and future risks. Protecting the privacy of genomic data is essential to maintain public trust and prevent misuse. Data breaches and unauthorized access to genomic data can have serious consequences, including discrimination, stigmatization, and emotional distress [24].
Strong data security measures are needed to protect genomic data from unauthorized access. These measures include encryption, access controls, and data anonymization techniques. Data governance frameworks should be established to ensure that genomic data is used responsibly and ethically. Individuals should have control over their own genomic data and should be able to decide who has access to it and how it is used [25].
4.2 Equity and Access
Ensuring equitable access to genomic technologies and the benefits they offer is a major challenge. The cost of genomic testing and personalized medicine can be prohibitive for many individuals, particularly those from low-income backgrounds and underserved communities. This can exacerbate existing health disparities and create new ones [26].
Efforts are needed to reduce the cost of genomic testing and personalized medicine and to ensure that these technologies are accessible to all individuals, regardless of their socioeconomic status or geographic location. Public funding for genomic research and clinical services can help to ensure equitable access. Outreach programs are needed to educate underserved communities about the benefits of genomics and to address their concerns about data privacy and genetic discrimination [27].
4.3 Genetic Discrimination
The potential for genetic discrimination is a major concern. Genetic information could be used by employers, insurers, or other entities to discriminate against individuals based on their genetic predispositions to disease [28]. This could lead to job loss, denial of insurance coverage, or other forms of discrimination.
Laws and regulations are needed to protect individuals from genetic discrimination. The Genetic Information Nondiscrimination Act (GINA) in the United States prohibits genetic discrimination in employment and health insurance [29]. However, GINA does not cover life insurance, long-term care insurance, or disability insurance. Stronger protections are needed to prevent genetic discrimination in all areas of life.
4.4 Incidental Findings
Genomic testing can sometimes reveal incidental findings, which are genetic variants that are unrelated to the primary purpose of the testing but may have implications for an individual’s health. For example, a genomic test performed to diagnose a genetic disease may reveal a variant that increases the individual’s risk of developing cancer [30].
The management of incidental findings raises ethical dilemmas. Should individuals be informed about incidental findings, even if they are not actionable or if the individual does not want to know? How should incidental findings be communicated to patients and their families? Clear guidelines are needed to address these issues and to ensure that incidental findings are managed responsibly and ethically.
Many thanks to our sponsor Esdebe who helped us prepare this research report.
5. Regulatory Challenges
The rapid pace of innovation in genomics has outpaced the development of regulations and guidelines to govern the use of genomic technologies. This has created uncertainty and challenges for researchers, clinicians, and industry stakeholders. Increasing regulatory scrutiny is apparent across the genomics field.
5.1 Regulation of Genomic Testing
The regulation of genomic testing varies widely across different countries and regions. In the United States, genomic testing is regulated by the Food and Drug Administration (FDA) and the Centers for Medicare & Medicaid Services (CMS) [31]. The FDA regulates the safety and efficacy of diagnostic tests, while CMS regulates the quality of laboratory testing.
Direct-to-consumer (DTC) genetic testing, which allows individuals to purchase genomic tests directly from companies without a physician’s order, raises particular regulatory challenges. The FDA has limited oversight of DTC genetic testing, and concerns have been raised about the accuracy, reliability, and clinical utility of these tests [32]. Clearer regulations are needed to ensure that DTC genetic tests are accurate, reliable, and provide meaningful information to consumers.
5.2 Data Governance and Security
The increasing volume of genomic data being generated and shared requires robust data governance and security frameworks. These frameworks should address issues such as data privacy, data access, data sharing, and data security. Data governance policies should be transparent and accountable, and they should be developed in consultation with stakeholders, including researchers, clinicians, patients, and industry representatives [33].
Data security measures should be implemented to protect genomic data from unauthorized access, theft, and misuse. These measures should include encryption, access controls, and data anonymization techniques. Regular audits and security assessments should be conducted to ensure that data security measures are effective.
5.3 Intellectual Property
Intellectual property (IP) rights, such as patents, can play a significant role in the development and commercialization of genomic technologies. However, overly broad or restrictive IP rights can hinder innovation and limit access to genomic technologies [34].
A balance is needed between protecting IP rights and promoting innovation and access. Patent laws should be carefully crafted to ensure that they do not stifle innovation or limit access to essential genomic technologies. Compulsory licensing or other mechanisms may be needed to ensure that essential genomic technologies are available to all individuals, regardless of their ability to pay.
Many thanks to our sponsor Esdebe who helped us prepare this research report.
6. Impact on Healthcare
Genomics is transforming healthcare in numerous ways, from improving disease diagnosis and treatment to enabling personalized medicine and preventing disease. The overall impact of genomics on healthcare is expected to be profound, leading to more effective, efficient, and equitable healthcare systems.
6.1 Improved Disease Diagnosis and Treatment
Genomic testing is improving disease diagnosis by identifying the underlying genetic causes of disease and by providing more accurate and timely diagnoses. This can lead to earlier intervention and more effective treatment. Genomic testing is also being used to predict treatment responses, allowing clinicians to tailor treatment to the individual characteristics of each patient [35].
6.2 Personalized Medicine
Personalized medicine, enabled by genomics, is transforming healthcare by tailoring medical treatment to the individual characteristics of each patient. This can lead to more effective treatment, fewer side effects, and improved patient outcomes. Personalized medicine is also being used to prevent disease by identifying individuals who are at risk for developing certain diseases and by providing them with personalized prevention strategies [36].
6.3 Disease Prevention
Genomics is playing an increasingly important role in disease prevention by identifying individuals who are at risk for developing certain diseases and by providing them with personalized prevention strategies. This can lead to a reduction in the incidence of disease and improved health outcomes. Genomic screening programs are being implemented to identify individuals who are at risk for developing certain cancers, heart disease, and other common diseases [37].
6.4 Public Health
Genomics is also having a significant impact on public health by improving our understanding of infectious diseases, monitoring the spread of antimicrobial resistance, and developing new vaccines and treatments. Genomic surveillance of viruses, such as SARS-CoV-2, has been crucial for understanding the evolution and transmission of the virus and for developing effective vaccines and treatments [38].
Many thanks to our sponsor Esdebe who helped us prepare this research report.
7. Conclusion
Genomics is a rapidly evolving field with tremendous potential to transform healthcare and other industries. Technological advancements in sequencing, data analysis, and genome editing are driving innovation and expanding the scope of genomic research and its applications. Genomics is already having a significant impact on disease diagnosis, personalized medicine, drug discovery, and disease prevention.
However, the rapid progress in genomics also raises significant ethical, legal, and social implications that demand careful consideration. Protecting data privacy, ensuring equitable access, preventing genetic discrimination, and managing incidental findings are critical challenges that must be addressed. Clear regulations and guidelines are needed to govern the use of genomic technologies and to ensure that they are used responsibly and ethically.
Future research and development efforts should focus on improving sequencing technologies, developing more sophisticated data analysis methods, enhancing the specificity and safety of genome editing technologies, and addressing the ethical and regulatory challenges associated with genomics. By addressing these challenges, we can unlock the full potential of genomics to improve human health and well-being.
References
[1] Venter, J. C., et al. “The sequence of the human genome.” Science 291.5507 (2001): 1304-1351.
[2] Illumina Sequencing Technology. (n.d.). Retrieved from https://www.illumina.com/science/technology/next-generation-sequencing/sequencing-technology.html
[3] Eid, J., et al. “Real-time DNA sequencing from single polymerase molecules.” Science 323.5910 (2009): 133-138.
[4] Deamer, D., et al. “Nanopore DNA sequencing.” Nature nanotechnology 11.1 (2016): 29-34.
[5] Tang, F., et al. “mRNA-Seq whole-transcriptome analysis of a single cell.” Nature methods 6.5 (2009): 377-382.
[6] Asp, M., et al. “Visium spatial gene expression reveals distinct molecular signatures in mouse brain.” Nature methods 17.3 (2020): 259-262.
[7] Libbrecht, M. W., & Noble, W. S. “Machine learning applications in genetics and genomics.” Nature Reviews Genetics 16.6 (2015): 321-332.
[8] Stein, L. D. “The case for cloud computing in genome informatics.” Genome biology 11.5 (2010): 207.
[9] Jinek, M., et al. “A programmable dual-RNA–guided DNA endonuclease in adaptive bacterial immunity.” Science 337.6096 (2012): 816-821.
[10] Hsu, P. D., et al. “DNA targeting specificity of RNA-guided Cas9 nucleases.” Nature biotechnology 31.9 (2013): 827-832.
[11] Anzalone, A. V., et al. “Search-and-replace genome editing without double-strand breaks or donor DNA.” Nature 576.7785 (2019): 149-157.
[12] Frangoul, H., et al. “CRISPR-Cas9 gene editing for sickle cell disease and β-thalassemia.” New England Journal of Medicine 384.3 (2021): 252-260.
[13] National Human Genome Research Institute. (n.d.). Genetic Testing. Retrieved from https://www.genome.gov/about-genomics/fact-sheets/Genetic-Testing
[14] Boycott, K. M., et al. “Rare-disease genetics in the era of next-generation sequencing: discovery, diagnosis, and translation.” The Lancet Neurology 12.7 (2013): 680-695.
[15] Didelot, X., et al. “Genomic epidemiology of bacterial infections, with a focus on public health.” The New England Journal of Medicine 377.26 (2017): 2602-2612.
[16] Garralda, E., et al. “Genomic analysis to select treatments for cancer: a systematic review and meta-analysis.” The Lancet Oncology 15.9 (2014): 1030-1042.
[17] National Research Council. (2011). Toward precision medicine: building a knowledge foundation for biomedical research and a new taxonomy of disease. National Academies Press.
[18] Relling, M. V., & Evans, W. E. “Pharmacogenomics in the clinic.” Nature 526.7573 (2015): 343-350.
[19] Sadee, W., & Dai, Z. “Pharmacogenomics/personalized medicine.” Human molecular genetics 14.suppl_2 (2005): R207-R214.
[20] Diamandis, E. P. “Personalized medicine: translating genomic biomarkers into patient-tailored therapeutics.” Expert opinion on biological therapy 13.4 (2013): 447-452.
[21] Varshney, R. K., et al. “Genomics approaches for crop improvement.” Plant cell reports 33.1 (2014): 1-17.
[22] Butler, J. M. “Advanced topics in forensic DNA typing: methodology.” Academic Press, 2011.
[23] Gilbert, J. A., & Neufeld, J. D. “Microbial ecology of the built environment.” Nature Reviews Microbiology 12.8 (2014): 588-594.
[24] Kaye, J., et al. “Data sharing in genomics–re-shaping scientific practice.” Nature Reviews Genetics 10.5 (2009): 331-335.
[25] McGuire, A. L., et al. “Genomic research and human subject protection: respecting the autonomy of individuals and protecting the interests of communities.” Genome research 18.6 (2008): 836-845.
[26] Khoury, M. J., et al. “Genomic medicine: from promise to population impact.” Genetics in Medicine 9.3 (2007): 139-148.
[27] Ashley, E. A. “Towards precision medicine.” The Lancet 386.9999 (2015): 1210-1222.
[28] Billings, P. R., et al. “Discrimination as a consequence of genetic testing.” American Journal of Human Genetics 50.3 (1992): 476.
[29] Genetic Information Nondiscrimination Act of 2008 (GINA). (n.d.). Retrieved from https://www.eeoc.gov/statutes/genetic-information-nondiscrimination-act-2008
[30] Berg, J. S., et al. “Recommendations for reporting of secondary findings in clinical exome and genome sequencing, 2021 update: a policy statement of the American College of Medical Genetics and Genomics (ACMG).” Genetics in Medicine 23.8 (2021): 1381-1388.
[31] U.S. Food and Drug Administration (FDA). (n.d.). Retrieved from https://www.fda.gov/
[32] Phillips, A. “Direct-to-consumer genetic testing: good, bad or benign?” Nature Reviews Genetics 12.10 (2011): 759-767.
[33] Shabani, M., et al. “The ELSI of genomic data sharing: a systematic review.” Genome medicine 3.5 (2011): 31.
[34] Cook-Deegan, R., & Heaney, C. “Patents in the genomic era.” The American Journal of Law & Medicine 34.1 (2008): 1-36.
[35] Hamburg, M. A., & Collins, F. S. “The path to personalized medicine.” New England Journal of Medicine 363.4 (2010): 301-304.
[36] Ginsburg, G. S., & Willard, H. F. “Genomic and personalized medicine: foundations and applications.” Translational research 154.6 (2009): 243-257.
[37] Janssens, A. C. J. W., et al. “Predictive genetic testing for multifactorial diseases: current perspectives.” Journal of medical genetics 43.5 (2006): 373-381.
[38] Rambaut, A., et al. “Preliminary genomic characterisation of an emergent SARS-CoV-2 lineage in the UK defined by a novel set of spike mutations.” Virological.org (2020).
This overview of regulatory challenges is insightful. Given the complexities of genomic data governance, I wonder if there’s a growing trend towards international collaborations to standardize regulations and data-sharing practices, especially considering the global nature of research and potential applications.
Thanks for your comment! You’ve highlighted a critical area. International collaboration is indeed gaining momentum. Standardizing regulations and data-sharing is crucial for the future of genomics, especially with research increasingly spanning across borders. Encouraging unified frameworks will ensure ethical and effective progress on a global scale.
Editor: MedTechNews.Uk
Thank you to our Sponsor Esdebe
This report effectively highlights the growing need for robust data infrastructure to manage the increasing volume of genomic data. Further discussion on advancements in data compression techniques and efficient storage solutions would be valuable to address this challenge.
Thanks for pointing out the need for advancements in data compression techniques. We agree that they are crucial. Exploring innovative methods like lossless compression algorithms and hardware acceleration for data processing could significantly reduce storage costs and improve data accessibility for researchers and clinicians.
Editor: MedTechNews.Uk
Thank you to our Sponsor Esdebe