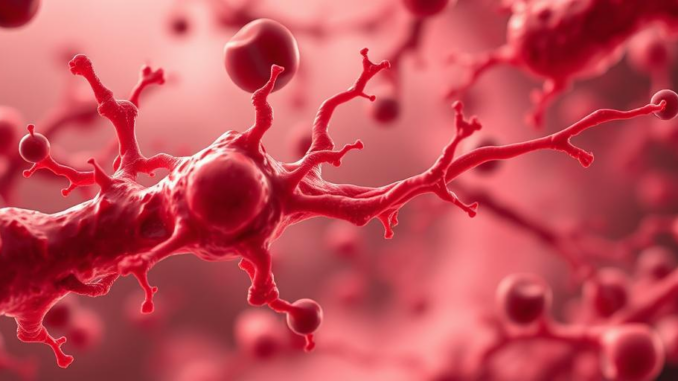
Abstract
Thromboinflammation describes the complex, bidirectional relationship between coagulation and inflammation. This intricate interplay significantly contributes to the pathogenesis of a wide range of diseases, including sepsis, cardiovascular diseases, autoimmune disorders, and cancer. While the ‘chip’ model provides a basic framework, the reality is far more nuanced, involving a multitude of cellular and molecular interactions. This review provides an in-depth exploration of the mechanisms underlying thromboinflammation, its multifaceted role in various diseases, existing and emerging therapeutic strategies, and the challenges that remain in translating research findings into clinical benefit. We examine the complex signalling pathways involved, the contribution of different immune cell subsets, and discuss the potential of targeting specific mediators to disrupt the thromboinflammatory cycle and improve patient outcomes. Finally, we highlight the need for a more holistic and personalized approach to understanding and treating thromboinflammation.
Many thanks to our sponsor Esdebe who helped us prepare this research report.
1. Introduction
Inflammation and coagulation, historically viewed as distinct physiological processes, are now recognized to be intimately intertwined. This interconnectedness, termed ‘thromboinflammation,’ describes the reciprocal relationship whereby inflammation drives coagulation activation and, conversely, coagulation pathways modulate inflammatory responses. While coagulation traditionally focused on hemostasis and thrombosis prevention, and inflammation on pathogen clearance and tissue repair, their simultaneous activation in various disease states highlights their role in driving disease progression. This understanding is crucial for developing more effective therapeutic strategies that target the underlying mechanisms of these complex conditions.
The recognition of thromboinflammation as a key driver of pathology has transformed our understanding of conditions ranging from acute infections like sepsis to chronic diseases such as atherosclerosis and cancer. The ‘chip’ model, a simplified representation of this process, posits that activated endothelial cells express Tissue Factor (TF), initiating the coagulation cascade and leading to thrombin generation. Thrombin, in turn, activates protease-activated receptors (PARs) on various cell types, including platelets and endothelial cells, further amplifying inflammation. While this model provides a useful starting point, the reality is significantly more complex and involves numerous other factors, including inflammatory cytokines, chemokines, reactive oxygen species (ROS), complement activation, and the coordinated action of diverse immune cell subsets.
This review aims to provide a comprehensive overview of thromboinflammation, moving beyond the limitations of simplified models to explore the intricate molecular and cellular mechanisms involved. We will delve into the role of thromboinflammation in specific disease contexts, discuss existing therapeutic approaches and novel drug targets, and highlight the challenges and future directions in this rapidly evolving field.
Many thanks to our sponsor Esdebe who helped us prepare this research report.
2. Mechanisms Underlying Thromboinflammation
The interplay between coagulation and inflammation is mediated by a complex network of cellular and molecular interactions. Understanding these mechanisms is critical for developing targeted therapies.
2.1. Tissue Factor and the Coagulation Cascade
Tissue Factor (TF) is a transmembrane glycoprotein that initiates the extrinsic pathway of the coagulation cascade upon exposure to blood. While TF is constitutively expressed by subendothelial cells and perivascular cells, its expression can be induced on endothelial cells, leukocytes, and cancer cells by inflammatory mediators such as TNF-α, IL-1β, and LPS. Following vascular injury or inflammation, TF binds to Factor VIIa, initiating a proteolytic cascade that ultimately leads to thrombin generation. Thrombin, the central enzyme of the coagulation cascade, not only converts fibrinogen to fibrin, forming a clot, but also exerts potent pro-inflammatory effects by activating protease-activated receptors (PARs) on various cell types.
2.2. Protease-Activated Receptors (PARs)
PARs are G protein-coupled receptors activated by proteolytic cleavage of their N-terminal extracellular domain. Thrombin is a major activator of PARs, particularly PAR1, PAR3, and PAR4. Activation of PARs triggers a wide range of cellular responses, including platelet activation, endothelial cell activation, increased vascular permeability, and the release of inflammatory mediators. PARs also play a role in leukocyte recruitment and activation, further amplifying the inflammatory response. The specific PAR subtypes expressed and the downstream signaling pathways activated vary depending on the cell type and the context, contributing to the complexity of thromboinflammation.
2.3. Platelets: More Than Just Clotting Cells
Platelets, traditionally known for their role in hemostasis, are increasingly recognized as key players in inflammation. Activated platelets release a plethora of inflammatory mediators, including chemokines (e.g., CCL5, CXCL4), cytokines (e.g., IL-1β), and growth factors (e.g., VEGF). These mediators recruit and activate leukocytes, promote endothelial cell activation, and contribute to the development of inflammation and tissue damage. Platelets also express adhesion molecules, such as P-selectin, which facilitate their interaction with leukocytes, forming platelet-leukocyte aggregates that further amplify the inflammatory response. Furthermore, platelets can directly interact with pathogens, modulating the immune response and contributing to the pathogenesis of infectious diseases.
2.4. Neutrophils: The First Responders
Neutrophils, the most abundant leukocytes in the circulation, are critical for host defense against infection and play a significant role in thromboinflammation. Upon activation, neutrophils release a variety of inflammatory mediators, including ROS, proteases (e.g., elastase), and cytokines (e.g., IL-8, TNF-α). They also undergo a unique form of cell death called NETosis, in which they release their DNA and histones into the extracellular space, forming Neutrophil Extracellular Traps (NETs). NETs can trap and kill pathogens, but they can also contribute to thrombosis and inflammation by activating coagulation factors, promoting platelet aggregation, and inducing endothelial cell damage. Dysregulated NET formation has been implicated in the pathogenesis of various thromboinflammatory diseases.
2.5. Endothelial Cells: Gatekeepers of the Vasculature
Endothelial cells, lining the inner surface of blood vessels, play a crucial role in regulating vascular permeability, leukocyte trafficking, and coagulation. During inflammation, endothelial cells become activated and express adhesion molecules, such as E-selectin, ICAM-1, and VCAM-1, which facilitate leukocyte adhesion and transmigration. They also produce inflammatory mediators, such as chemokines, cytokines, and prostaglandins, contributing to the inflammatory response. Furthermore, activated endothelial cells can express TF, initiating the coagulation cascade and promoting thrombus formation. Endothelial dysfunction is a hallmark of thromboinflammatory diseases, leading to increased vascular permeability, leukocyte infiltration, and thrombosis.
2.6. The Complement System
The complement system, a crucial component of the innate immune system, plays a complex role in thromboinflammation. Complement activation can be triggered by both coagulation factors and inflammatory mediators, leading to the generation of complement fragments, such as C3a and C5a. These fragments act as potent anaphylatoxins, recruiting and activating leukocytes, increasing vascular permeability, and promoting inflammation. Complement activation can also directly activate platelets and endothelial cells, contributing to thrombosis. However, the complement system can also promote clot resolution and tissue repair, highlighting its dual role in thromboinflammation.
2.7. Cytokines and Chemokines: Orchestrators of the Inflammatory Response
Cytokines and chemokines are small signaling proteins that play a central role in regulating the inflammatory response. Pro-inflammatory cytokines, such as TNF-α, IL-1β, and IL-6, activate endothelial cells, induce TF expression, and promote leukocyte recruitment. Chemokines, such as CXCL8 (IL-8) and CCL2 (MCP-1), attract leukocytes to the site of inflammation. The dysregulation of cytokine and chemokine production is a hallmark of thromboinflammatory diseases, leading to a self-perpetuating cycle of inflammation and thrombosis.
Many thanks to our sponsor Esdebe who helped us prepare this research report.
3. Thromboinflammation in Specific Diseases
Thromboinflammation is implicated in the pathogenesis of a wide range of diseases, contributing to disease severity and mortality. Understanding the specific role of thromboinflammation in each disease is essential for developing targeted therapies.
3.1. Sepsis
Sepsis, a life-threatening condition caused by a dysregulated host response to infection, is a prime example of thromboinflammation. The systemic inflammatory response in sepsis leads to widespread endothelial cell activation, increased TF expression, and dysregulation of the coagulation cascade. This results in disseminated intravascular coagulation (DIC), characterized by widespread microvascular thrombosis and consumption of coagulation factors, leading to bleeding complications. Neutrophils and NETs play a critical role in sepsis-associated thromboinflammation, contributing to endothelial damage and thrombosis. The complement system is also activated, further amplifying the inflammatory response and contributing to organ dysfunction. Targeting specific mediators of thromboinflammation, such as TF, thrombin, and NETs, has shown promise in preclinical studies and clinical trials, but further research is needed to identify effective therapies.
3.2. Cardiovascular Diseases
Thromboinflammation plays a critical role in the pathogenesis of cardiovascular diseases, including atherosclerosis, myocardial infarction, and stroke. In atherosclerosis, chronic inflammation of the arterial wall promotes plaque formation and instability. Activated endothelial cells, leukocytes, and platelets contribute to the inflammatory process, leading to the recruitment of monocytes and T cells into the arterial wall. These cells release inflammatory mediators that promote lipid accumulation, smooth muscle cell proliferation, and extracellular matrix remodeling. Plaque rupture triggers thrombus formation, leading to acute myocardial infarction or stroke. Thromboinflammation also contributes to the development of heart failure, through mechanisms involving cardiomyocyte inflammation and fibrosis. Targeting inflammatory pathways in cardiovascular disease, such as IL-1β, has shown promise in clinical trials.
3.3. Autoimmune Diseases
Autoimmune diseases, characterized by aberrant immune responses against self-antigens, are often associated with thromboinflammation. In diseases such as systemic lupus erythematosus (SLE) and rheumatoid arthritis (RA), chronic inflammation leads to endothelial cell activation, increased TF expression, and dysregulation of the coagulation cascade. Autoantibodies against phospholipids, such as those found in antiphospholipid syndrome (APS), can activate endothelial cells and platelets, promoting thrombosis. NETs have also been implicated in the pathogenesis of autoimmune diseases, contributing to inflammation and tissue damage. Targeting specific mediators of thromboinflammation, such as TNF-α, IL-6, and B cells, has been shown to be effective in treating autoimmune diseases, but further research is needed to address the thromboinflammatory component.
3.4. Cancer
Cancer and thrombosis are closely linked, with cancer patients having a significantly increased risk of venous thromboembolism (VTE). Cancer cells can directly activate the coagulation cascade by expressing TF and releasing procoagulant factors. They can also stimulate endothelial cells and leukocytes to release inflammatory mediators, promoting thrombosis. Furthermore, cancer cells can interact with platelets, promoting their activation and aggregation. Thromboinflammation can also contribute to cancer progression by promoting angiogenesis, metastasis, and immune evasion. Targeting specific mediators of thromboinflammation, such as TF, thrombin, and VEGF, has shown promise in preclinical studies and clinical trials, but further research is needed to develop effective therapies for cancer-associated thrombosis.
3.5. Sickle Cell Disease
Sickle Cell Disease (SCD) is an inherited blood disorder that results in chronic inflammation, vaso-occlusion, and end-organ damage. The abnormal hemoglobin (HbS) polymerizes under hypoxic conditions, causing red blood cells to become rigid and sickle-shaped. These sickle cells adhere to the endothelium, activate leukocytes, and promote thrombus formation. Chronic inflammation, driven by vaso-occlusion and hemolysis, contributes to endothelial dysfunction and increased TF expression. Platelets, neutrophils, and NETs also play a critical role in SCD-associated thromboinflammation. Targeting specific mediators of thromboinflammation, such as P-selectin and adhesion molecules, has shown promise in preclinical studies and clinical trials for SCD.
Many thanks to our sponsor Esdebe who helped us prepare this research report.
4. Therapeutic Strategies for Targeting Thromboinflammation
Targeting thromboinflammation represents a promising therapeutic strategy for a wide range of diseases. Existing and emerging therapies aim to disrupt the interplay between coagulation and inflammation.
4.1. Anticoagulants
Anticoagulants, such as heparin, warfarin, and direct oral anticoagulants (DOACs), are commonly used to prevent and treat thrombosis. Heparin inhibits thrombin and other coagulation factors, while warfarin inhibits vitamin K-dependent coagulation factors. DOACs directly inhibit thrombin (e.g., dabigatran) or Factor Xa (e.g., rivaroxaban, apixaban). While anticoagulants are effective in preventing thrombosis, they do not directly target the inflammatory component of thromboinflammation. However, some anticoagulants, such as heparin, have been shown to have anti-inflammatory effects, possibly by inhibiting complement activation and leukocyte adhesion.
4.2. Antiplatelet Agents
Antiplatelet agents, such as aspirin and clopidogrel, inhibit platelet activation and aggregation. Aspirin inhibits cyclooxygenase-1 (COX-1), reducing the production of thromboxane A2, a potent platelet activator. Clopidogrel inhibits the P2Y12 receptor, blocking ADP-mediated platelet activation. Antiplatelet agents are effective in preventing arterial thrombosis, but they do not directly target the inflammatory component of thromboinflammation. However, platelets are key players in inflammation, and antiplatelet agents can reduce inflammation by inhibiting platelet-mediated leukocyte activation and cytokine release.
4.3. Anti-inflammatory Therapies
Anti-inflammatory therapies, such as corticosteroids, TNF-α inhibitors, and IL-1β inhibitors, target the inflammatory component of thromboinflammation. Corticosteroids are broad-spectrum anti-inflammatory agents that inhibit the production of various inflammatory mediators. TNF-α inhibitors, such as infliximab and etanercept, block the activity of TNF-α, a key pro-inflammatory cytokine. IL-1β inhibitors, such as anakinra and canakinumab, block the activity of IL-1β, another key pro-inflammatory cytokine. These therapies have been shown to be effective in treating autoimmune diseases and inflammatory conditions, but they may have limited efficacy in preventing thrombosis.
4.4. Targeting NETs
Given the crucial role of NETs in thromboinflammation, targeting NET formation or disrupting their activity represents a promising therapeutic strategy. DNase I, an enzyme that degrades DNA, can dissolve NETs and reduce thrombosis and inflammation. Other strategies include inhibiting PAD4, an enzyme required for NET formation, or blocking the interaction of NETs with coagulation factors and platelets. Several preclinical studies have shown that targeting NETs can be effective in treating thromboinflammatory diseases, but further research is needed to translate these findings into clinical benefit.
4.5. Novel Therapeutic Targets
Several novel therapeutic targets for thromboinflammation are currently under investigation. These include:
- Tissue Factor (TF) inhibitors: Inhibiting TF activity can prevent the initiation of the coagulation cascade and reduce thrombin generation.
- Protease-Activated Receptor (PAR) antagonists: Blocking PAR activation can inhibit the pro-inflammatory effects of thrombin.
- Adhesion molecule inhibitors: Inhibiting the interaction of leukocytes with endothelial cells can reduce leukocyte recruitment and inflammation.
- Complement inhibitors: Blocking complement activation can reduce inflammation and thrombosis.
- MicroRNA-based therapies: Targeting specific microRNAs that regulate coagulation and inflammation can modulate the thromboinflammatory response.
Many thanks to our sponsor Esdebe who helped us prepare this research report.
5. Challenges and Future Directions
Despite significant progress in understanding thromboinflammation, several challenges remain in translating research findings into clinical benefit. These challenges include:
- Complexity of the thromboinflammatory response: Thromboinflammation is a complex process involving numerous cellular and molecular interactions. Understanding the specific mechanisms involved in each disease is essential for developing targeted therapies.
- Heterogeneity of patient populations: Patients with thromboinflammatory diseases are a heterogeneous group, with varying disease severity and underlying conditions. Identifying biomarkers that predict treatment response is crucial for personalized therapy.
- Lack of specific and effective therapies: Existing therapies for thromboinflammation often have limited efficacy and significant side effects. Developing more specific and effective therapies is a major priority.
- Translational challenges: Preclinical studies of thromboinflammation often fail to translate into clinical benefit. Improving the design of preclinical studies and using more relevant animal models is essential.
Future research directions in thromboinflammation include:
- Identifying novel therapeutic targets: Exploring the intricate molecular and cellular mechanisms of thromboinflammation to identify new targets for therapeutic intervention.
- Developing personalized therapies: Identifying biomarkers that predict treatment response and tailoring therapy to individual patients.
- Improving preclinical models: Developing more relevant animal models that accurately reflect human thromboinflammatory diseases.
- Conducting large-scale clinical trials: Evaluating the efficacy and safety of novel therapies in large-scale clinical trials.
- Investigating the long-term consequences of thromboinflammation: Studying the long-term effects of thromboinflammation on organ function and overall health.
Many thanks to our sponsor Esdebe who helped us prepare this research report.
6. Conclusion
Thromboinflammation is a complex and multifaceted process that plays a critical role in the pathogenesis of a wide range of diseases. Understanding the intricate interplay between coagulation and inflammation is essential for developing effective therapeutic strategies. While existing therapies, such as anticoagulants, antiplatelet agents, and anti-inflammatory therapies, can be effective in some cases, they often have limited efficacy and significant side effects. Novel therapeutic targets, such as TF, PARs, NETs, and complement, are currently under investigation and hold promise for the treatment of thromboinflammatory diseases. Future research efforts should focus on identifying novel therapeutic targets, developing personalized therapies, improving preclinical models, and conducting large-scale clinical trials. A more holistic and personalized approach to understanding and treating thromboinflammation is needed to improve patient outcomes and reduce the burden of these complex and life-threatening diseases.
Many thanks to our sponsor Esdebe who helped us prepare this research report.
References
- Esmon, C. T. (2004). The interactions between inflammation and coagulation. British Journal of Haematology, 126(4), 513-520.
- Levi, M., van der Poll, T., & Büller, H. R. (2004). Bidirectional relation between inflammation and coagulation. Circulation, 109(22), 2698-2704.
- Engelmann, B., Brandl, R., Poggi, M., & Renner, N. (2011). Thromboinflammation in cardiovascular disease. Thrombosis and Haemostasis, 105(06), 980-992.
- Crouser, E. D. (2020). Pathophysiology of sepsis-induced organ dysfunction. Critical Care Clinics, 36(2), 237-264.
- Kaplan, M. J., & Radu, A. L. (2011). Neutrophil extracellular traps: double-edged swords of immunity. Nature Reviews Rheumatology, 7(9), 521-529.
- Ammollo, T., Semeraro, F., Xu, J., Di Santo, A., Bastianelli, P., Romoli, D., … & Semeraro, N. (2011). Role of microparticles in disseminated intravascular coagulation during sepsis. Critical Care Medicine, 39(6), 1434-1441.
- Rondeau, E., & Veyradier, A. (2016). Thrombotic microangiopathies. Nephrology Dialysis Transplantation, 31(9), 1426-1434.
- Libby, P., Ridker, P. M., & Hansson, G. K. (2011). Inflammation in atherosclerosis. Nature, 473(7347), 317-325.
- Ridker, P. M., Everett, B. M., Thuren, T., MacFadyen, J., Chang, W. H., Ballantyne, C., … & Fonseca, F. (2017). Antiinflammatory therapy with canakinumab for atherosclerotic disease. New England Journal of Medicine, 377(12), 1119-1131.
- Knight, J. S., Carmona-Rivera, C., Shupe, J., & Kaplan, M. J. (2012). Neutrophil extracellular traps drive thrombosis in lupus. Annals of the Rheumatic Diseases, 71(12), 2019-2027.
- Rickles, F. R., & Falanga, A. (2001). Molecular mechanisms of the relationship between thrombosis and cancer. Thrombosis Research, 102(6), V215-V224.
- Hisada, Y., Geddings, J. E., & Kim, P. Y. (2018). Hemostasis and thrombosis in sickle cell disease: a multifaceted vasculopathy. Journal of Thrombosis and Haemostasis, 16(6), 895-906.
- Brandão, T. A., de Souza, M. G., & Albuquerque, D. M. (2020). The role of P-selectin in sickle cell disease vaso-occlusion: evidence from experimental and clinical studies. Frontiers in Physiology, 11, 579038.
- Esmon, C. T. (2005). Inflammation and thrombosis. Haemostasis, 35(1-2), 30-35.
- Khandpur, R., Carmona-Rivera, C., & Kaplan, M. J. (2022). NETs and thromboinflammation in systemic autoimmune diseases. Clinical Immunology, 244, 109116.
- Thålin, C. R., Hisada, Y., Lundström, S., Mackman, N., Dahlbäck, B., Wagner, D. D., … & Borén, J. (2016). Enhanced tissue factor-dependent coagulation in transgenic mice expressing human C-reactive protein. Journal of Thrombosis and Haemostasis, 14(3), 559-568.
- Vögele, M., & von Bruhl, M. L. (2023). Neutrophil extracellular traps in thromboinflammation and disease. Seminars in Immunopathology, 45(5), 611-622.
The discussion of NETs as therapeutic targets is particularly compelling. What are the latest insights into the potential of modulating NET formation or degradation in specific disease contexts, particularly concerning off-target effects and long-term consequences?
Thanks for highlighting NETs! It’s a hot area. Current research explores targeted inhibition of NET formation via PAD4 inhibitors to minimize off-target effects. Studies are also investigating long-term impacts of NET modulation on immune function and tissue repair processes across various thromboinflammatory conditions. It’s complex, but promising!
Editor: MedTechNews.Uk
Thank you to our Sponsor Esdebe