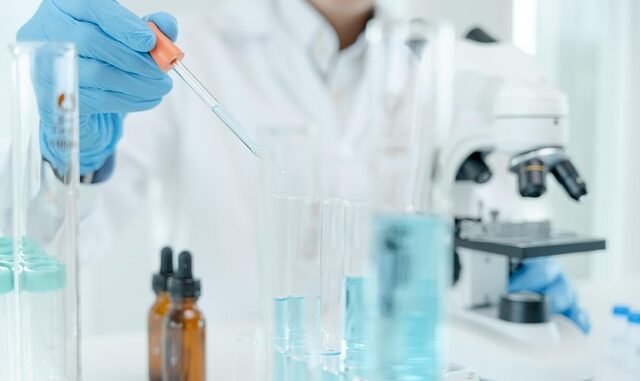
Abstract
Chromothripsis, a catastrophic genomic event involving massive chromosomal rearrangements, has emerged as a significant factor in cancer development and other diseases. Unlike gradual accumulation of mutations, chromothripsis involves a single, abrupt event leading to complex structural variations, including deletions, inversions, duplications, and translocations within a localized region. This review comprehensively explores the multifaceted nature of chromothripsis, dissecting its proposed mechanisms, expanding its prevalence beyond its initial association with acute myeloid leukemia (AML) and congenital disorders like Lamb-Shaffer Syndrome (LGS), detailing current detection methodologies, and discussing potential therapeutic interventions. Furthermore, it critically examines the elusive causes of chromothripsis, focusing on potential triggers and predisposing factors within specific individuals or cell types. The convergence of advanced genomic technologies and mechanistic insights offers promising avenues for targeted therapeutic strategies and a deeper understanding of genome instability.
Many thanks to our sponsor Esdebe who helped us prepare this research report.
1. Introduction
The conventional model of tumorigenesis posits that cancer develops through the gradual accumulation of genetic mutations over time. However, the discovery of chromothripsis has challenged this linear progression, revealing a mechanism by which a single catastrophic event can instantaneously reshape the genome. Chromothripsis, derived from the Greek words for “chromosome shattering,” describes a process in which a chromosome undergoes fragmentation followed by seemingly random reassembly, resulting in complex and localized rearrangements. This phenomenon was initially observed in cancer cells and later implicated in various congenital disorders, highlighting its potential to drive both somatic and germline mutations [1]. While much research has focused on its implications in leukemia, emerging studies suggest a broader role for chromothripsis in solid tumors, developmental disorders, and even aging-related processes. The exact mechanisms underlying chromothripsis remain debated, with several models proposed, each attempting to explain the observed patterns of chromosomal rearrangements. The detection of chromothripsis relies heavily on advanced genomic technologies, including next-generation sequencing (NGS) and array-based comparative genomic hybridization (aCGH), which provide high-resolution views of chromosomal architecture. Furthermore, the development of computational algorithms capable of identifying characteristic chromothripsis signatures from genomic data has become crucial. Given the potentially devastating consequences of chromothripsis, there is a growing need to develop therapeutic strategies targeting its underlying mechanisms or mitigating its effects. This review aims to provide a comprehensive overview of chromothripsis, encompassing its mechanisms, prevalence, detection methods, etiological factors, and potential therapeutic interventions. The broader perspective allows for a more complete understanding of how chromothripsis influences genome stability and disease development.
Many thanks to our sponsor Esdebe who helped us prepare this research report.
2. Mechanisms of Chromothripsis: Shattering and Reassembly
The precise molecular mechanisms driving chromothripsis remain a subject of intense investigation. Several models have been proposed, each with supporting evidence, but none fully explain all aspects of the phenomenon. A critical aspect of chromothripsis is the confinement of rearrangements to one or a few chromosomes, suggesting a spatially restricted event. Here, we delve into the most prominent models:
2.1. The Micronucleus Model
The micronucleus (MN) model is one of the most widely accepted explanations for chromothripsis. Micronuclei are small, extranuclear bodies that form during cell division when chromosome fragments or whole chromosomes lag behind and fail to be incorporated into the main nucleus. These MNs are prone to DNA damage and replication stress due to their abnormal structure and delayed replication timing [2]. Furthermore, the nuclear envelope of the micronucleus is more prone to rupture than the main nucleus allowing the ingress of DNA degrading enzymes such as DNAses. This can lead to shattering of the chromosome within the MN. Subsequent incorporation of the fragmented DNA back into the main nucleus during the next cell division can result in the complex rearrangements characteristic of chromothripsis. Evidence supporting this model includes the observation of MN formation in cells undergoing chromothripsis and the identification of MN-specific DNA damage signatures within chromothriptic regions [3]. However, some studies challenge this model, suggesting that MN formation may be a consequence rather than a cause of chromothripsis.
2.2. Replication Catastrophe
Replication stress, characterized by stalled or collapsed replication forks, can trigger massive DNA damage and chromosomal instability. In the context of chromothripsis, replication catastrophe posits that a single instance of severe replication stress can lead to the fragmentation of a chromosome. This fragmentation could be further exacerbated by the activation of DNA damage checkpoints and the subsequent attempts at DNA repair [4]. Incompletely replicated regions are vulnerable to breakage, and stalled replication forks can collapse, resulting in double-strand breaks (DSBs). Error-prone repair mechanisms, such as non-homologous end joining (NHEJ), can then join these fragments in a haphazard manner, leading to the complex rearrangements observed in chromothripsis. The evidence is compelling for this mechanism as some chromothriptic events are associated with common fragile sites and regions of the genome that are difficult to replicate.
2.3. Abortive Apoptosis
Apoptosis, or programmed cell death, is a crucial cellular process that eliminates damaged or unwanted cells. However, when apoptosis is initiated but fails to complete properly, it can lead to DNA fragmentation. Abortive apoptosis proposes that incomplete execution of apoptosis results in chromosome shattering, and the subsequent DNA repair processes attempt to salvage the fragmented DNA, leading to chaotic rearrangements. DNase activity during apoptosis is tightly regulated to avoid unnecessary genomic damage. However, dysregulation of these DNases or premature termination of the apoptotic process could result in uncontrolled DNA fragmentation [5]. Furthermore, the repair mechanisms engaged after abortive apoptosis might be error-prone, contributing to the randomness of the rearrangements. This model offers a potential explanation for the presence of cell death-related signatures in chromothriptic regions and the observed association between chromothripsis and disrupted apoptotic pathways. It is also worth noting that these mechanisms are not mutually exclusive, and more than one may contribute to chromothripsis. For example, the initial insult to the chromosome may come from a replication error which then causes the creation of micronuclei which results in chromothripsis.
2.4. Chromosome Bridging
Chromosome bridges are formed when sister chromatids fail to fully separate during cell division, resulting in a physical connection between the dividing cells. The resolution of these bridges often involves chromosome breakage, which can lead to chromothripsis if the broken ends are reassembled incorrectly. Bridge-fusion-breakage (BFB) cycles contribute significantly to this process. Telomere dysfunction and dicentric chromosome formation are common drivers of BFB cycles [6]. The iterative breakage and fusion of chromosome arms eventually culminate in highly rearranged chromosomes. The BFB model is especially relevant in cancer cells with dysfunctional telomeres and defective DNA repair pathways. Chromosome bridging can also be caused by catenation between sister chromatids after replication. If the cell attempts to move into anaphase before the sister chromatids are fully separated this can cause breakage.
Many thanks to our sponsor Esdebe who helped us prepare this research report.
3. Prevalence of Chromothripsis in Diverse Diseases
While initially associated with specific cancers and congenital disorders, chromothripsis has now been identified in a wide range of diseases, suggesting a broader impact on genome instability and disease development. Here, we explore the prevalence of chromothripsis in various disease contexts.
3.1. Cancer
Chromothripsis is particularly prevalent in cancer, with varying frequencies depending on the cancer type. It is frequently observed in bone cancers such as osteosarcoma (up to 25%) and is implicated in promoting tumor progression and drug resistance [7]. In leukemia, chromothripsis has been found in both acute myeloid leukemia (AML) and chronic lymphocytic leukemia (CLL), contributing to the activation of oncogenes and the inactivation of tumor suppressor genes. Solid tumors such as prostate cancer, lung cancer, and colorectal cancer also exhibit chromothripsis, although at lower frequencies compared to some hematological malignancies [8]. In these cases, chromothripsis can drive the formation of complex genomic rearrangements that contribute to tumor heterogeneity and therapy resistance. The role of chromothripsis in cancer is often context-dependent, with specific genes and pathways affected by the rearrangements influencing the tumor phenotype and response to treatment.
3.2. Congenital Disorders
Chromothripsis has been identified as a causative factor in various congenital disorders, often presenting with complex phenotypes. Lamb-Shaffer Syndrome (LGS), characterized by intellectual disability, developmental delay, and distinctive facial features, was among the first congenital disorders linked to chromothripsis [9]. Chromothripsis in LGS typically involves rearrangements on chromosome 12q13, disrupting the MED12 gene. Other congenital disorders associated with chromothripsis include those with developmental abnormalities, neurological disorders, and multiple congenital anomalies. The detection of chromothripsis in these disorders has improved diagnostic capabilities and provided insights into the genetic basis of complex developmental syndromes. Often, chromothripsis leads to the deletion of many genes, which can result in a more severe phenotype than might be expected from a single point mutation in a critical gene.
3.3. Other Diseases and Conditions
Emerging evidence suggests that chromothripsis may also play a role in other diseases and conditions beyond cancer and congenital disorders. For example, chromothripsis has been implicated in some cases of infertility and pregnancy loss, suggesting that genomic instability in germ cells can lead to reproductive failures [10]. Furthermore, there is increasing interest in the potential role of chromothripsis in aging and age-related diseases. Accumulation of DNA damage and genomic instability are hallmarks of aging, and chromothripsis could contribute to this process by causing large-scale genomic rearrangements that disrupt cellular function. The extent to which chromothripsis contributes to these conditions remains an area of active investigation, but these initial findings suggest a broader role for chromothripsis in human health and disease.
Many thanks to our sponsor Esdebe who helped us prepare this research report.
4. Techniques for Detecting Chromothripsis
Detecting chromothripsis requires advanced genomic technologies capable of resolving complex chromosomal rearrangements at high resolution. Several techniques have been developed to identify chromothripsis events, each with its strengths and limitations.
4.1. Next-Generation Sequencing (NGS)
NGS has emerged as the primary tool for detecting chromothripsis due to its ability to provide comprehensive and high-resolution genomic data. Whole-genome sequencing (WGS) allows for the unbiased detection of structural variations, including deletions, duplications, inversions, and translocations, which are characteristic of chromothripsis [11]. By analyzing the pattern of breakpoint junctions and copy number alterations, it is possible to identify regions of the genome that have undergone chromothripsis. Targeted sequencing approaches, such as exome sequencing or gene panel sequencing, can also be used to detect chromothripsis in specific genomic regions of interest. However, these approaches may miss chromothripsis events that occur outside the targeted regions. The bioinformatics analysis of NGS data is crucial for identifying chromothripsis signatures. Algorithms have been developed to detect clustered breakpoints, oscillating copy number profiles, and other features indicative of chromothripsis.
4.2. Array-Based Comparative Genomic Hybridization (aCGH)
aCGH is a microarray-based technique that measures copy number variations across the genome. While aCGH does not provide the same resolution as NGS, it can still be useful for detecting large-scale deletions and duplications associated with chromothripsis [12]. aCGH involves hybridizing differentially labeled DNA from a sample and a reference to a microarray containing probes that represent different regions of the genome. The relative intensities of the two labels indicate copy number differences between the sample and the reference. In the context of chromothripsis, aCGH can reveal the oscillating copy number patterns that are characteristic of this phenomenon. However, aCGH is limited in its ability to detect balanced rearrangements such as inversions and translocations, which are also common features of chromothripsis.
4.3. Fluorescence In Situ Hybridization (FISH)
FISH is a cytogenetic technique that uses fluorescently labeled DNA probes to visualize specific DNA sequences on chromosomes. FISH can be used to confirm and refine the breakpoints identified by NGS or aCGH [13]. By using probes that span the breakpoints, it is possible to visualize the rearranged chromosomes and confirm the presence of complex structural variations. FISH is particularly useful for validating chromothripsis events in individual cells, providing insights into the heterogeneity of genomic rearrangements within a population of cells. However, FISH is a targeted approach that requires prior knowledge of the genomic regions of interest, limiting its ability to detect novel chromothripsis events.
4.4. Optical Genome Mapping (OGM)
Optical genome mapping is an emerging technology that provides long-range structural information about the genome. OGM involves labeling DNA molecules with fluorescent markers and imaging them as they pass through a nanochannel. This allows for the construction of a genome-wide map that reveals the arrangement of DNA sequences. OGM is particularly well-suited for detecting complex structural variations, including those associated with chromothripsis, as it can span large genomic regions and resolve breakpoints with high accuracy. Furthermore, OGM does not require PCR amplification, which can introduce bias into NGS data [14]. OGM is becoming an increasingly valuable tool for studying chromothripsis, particularly in cases where NGS data is difficult to interpret.
Many thanks to our sponsor Esdebe who helped us prepare this research report.
5. Etiology of Chromothripsis: Causes and Predisposing Factors
The causes of chromothripsis remain largely unknown, but several factors have been implicated in triggering this catastrophic event. Understanding the etiology of chromothripsis is crucial for developing strategies to prevent or mitigate its occurrence.
5.1. DNA Damage and Repair Defects
DNA damage is a major driver of genomic instability, and defects in DNA repair pathways can increase the susceptibility to chromothripsis. Cells with impaired DNA repair mechanisms are more likely to accumulate DNA damage, leading to chromosome fragmentation and rearrangements. Mutations in genes involved in DNA repair, such as BRCA1, BRCA2, and TP53, have been associated with increased risk of chromothripsis [15]. These genes play critical roles in repairing double-strand breaks and maintaining genome stability. When these genes are mutated or inactivated, cells become more vulnerable to DNA damage and chromosomal rearrangements. Furthermore, exposure to DNA-damaging agents, such as radiation and chemotherapy, can also increase the risk of chromothripsis. These agents can induce DNA breaks and other types of DNA damage, which can trigger the catastrophic events that lead to chromothripsis.
5.2. Telomere Dysfunction
Telomeres are protective caps at the ends of chromosomes that prevent DNA degradation and maintain genome stability. Telomere dysfunction, characterized by telomere shortening or loss of telomere capping, can lead to chromosome fusions and rearrangements. Cells with dysfunctional telomeres are prone to enter into breakage-fusion-bridge (BFB) cycles, which can result in complex genomic rearrangements, including chromothripsis [16]. Telomere dysfunction can be caused by various factors, including aging, genetic mutations, and environmental exposures. Mutations in genes involved in telomere maintenance, such as TERT and TERC, have been associated with increased risk of telomere dysfunction and chromothripsis. Furthermore, chronic inflammation and oxidative stress can also contribute to telomere shortening and dysfunction.
5.3. Chromatin Organization and Nuclear Architecture
The organization of chromatin within the nucleus plays a crucial role in maintaining genome stability and regulating gene expression. Disruption of chromatin organization can lead to increased DNA damage and chromosomal rearrangements. Chromatin is organized into topologically associating domains (TADs), which are discrete genomic regions that interact with each other more frequently than with other regions of the genome. Disruption of TAD boundaries can lead to aberrant interactions between different genomic regions, increasing the risk of chromosomal rearrangements [17]. Furthermore, the spatial arrangement of chromosomes within the nucleus can also influence the risk of chromothripsis. Chromosomes that are located in close proximity to each other are more likely to undergo rearrangements. Changes in nuclear architecture, such as alterations in nuclear shape and size, can also contribute to genome instability and chromothripsis. Mutations in genes involved in chromatin organization and nuclear architecture have been associated with increased risk of chromothripsis.
5.4. Environmental Factors
Environmental factors, such as exposure to toxins and infectious agents, can also contribute to chromothripsis. Exposure to certain chemicals, such as benzene and arsenic, has been linked to increased risk of chromosomal rearrangements and cancer. These chemicals can induce DNA damage and disrupt DNA repair pathways, increasing the susceptibility to chromothripsis [18]. Furthermore, infection with certain viruses, such as human papillomavirus (HPV) and Epstein-Barr virus (EBV), has been associated with increased risk of chromothripsis. These viruses can integrate into the host genome and disrupt DNA replication and repair, leading to chromosomal rearrangements.
Many thanks to our sponsor Esdebe who helped us prepare this research report.
6. Therapeutic Strategies Targeting Chromothripsis
Given the potentially devastating consequences of chromothripsis, there is a growing need to develop therapeutic strategies targeting its underlying mechanisms or mitigating its effects. Several approaches are being explored, ranging from preventing chromothripsis from occurring to selectively targeting cells that have undergone chromothripsis.
6.1. Prevention Strategies
Preventing chromothripsis from occurring in the first place is the most desirable therapeutic strategy. This can be achieved by reducing DNA damage, improving DNA repair, and maintaining telomere integrity. Lifestyle modifications, such as avoiding exposure to toxins and maintaining a healthy diet, can help to reduce DNA damage. Furthermore, chemoprevention strategies, such as using antioxidants and anti-inflammatory agents, can help to protect against DNA damage. Improving DNA repair can be achieved by targeting specific DNA repair pathways with small molecule inhibitors or gene therapy. For example, inhibiting PARP (poly ADP-ribose polymerase) can enhance the sensitivity of cancer cells with BRCA1/2 mutations to DNA-damaging agents. Maintaining telomere integrity can be achieved by promoting telomere elongation and preventing telomere shortening. This can be achieved by using telomerase activators or by targeting telomere dysfunction with small molecule inhibitors [19].
6.2. Selective Targeting of Chromothriptic Cells
Another therapeutic strategy is to selectively target cells that have undergone chromothripsis. This can be achieved by exploiting the unique vulnerabilities of these cells. Chromothriptic cells often exhibit increased DNA damage and replication stress, making them more sensitive to DNA-damaging agents. Furthermore, chromothriptic cells may have altered metabolic pathways, making them more vulnerable to metabolic inhibitors. Targeting specific genes or pathways that are disrupted by chromothripsis can also be an effective therapeutic strategy. For example, if chromothripsis leads to the activation of an oncogene, targeting that oncogene with a small molecule inhibitor can selectively kill chromothriptic cells. Furthermore, immunotherapy approaches, such as CAR-T cell therapy, can be used to target chromothriptic cells that express unique surface markers. For instance, it may be possible to target chromothriptic cancer cells that express neoantigens generated by the rearrangements.
6.3. Genome Editing Approaches
Genome editing technologies, such as CRISPR-Cas9, offer the potential to correct or mitigate the effects of chromothripsis. CRISPR-Cas9 can be used to precisely target specific DNA sequences and introduce targeted mutations or deletions. In the context of chromothripsis, CRISPR-Cas9 could be used to disrupt oncogenes that have been activated by the rearrangements or to repair tumor suppressor genes that have been inactivated. Furthermore, CRISPR-Cas9 could be used to correct the breakpoints of chromothriptic rearrangements, restoring the normal chromosomal structure. However, the use of CRISPR-Cas9 for therapeutic purposes is still in its early stages, and there are several challenges that need to be addressed, including off-target effects and delivery limitations. It may also be possible to use gene editing technologies to correct some of the damage caused by chromothripsis, although this is likely to be challenging given the large number of rearrangements that typically occur. The possibility to use genome editing approaches is also limited as in many cases the genome will be too damaged.
Many thanks to our sponsor Esdebe who helped us prepare this research report.
7. Future Directions and Concluding Remarks
Chromothripsis represents a paradigm shift in our understanding of genome instability and disease development. While significant progress has been made in elucidating the mechanisms, prevalence, detection, and etiology of chromothripsis, many questions remain unanswered. Future research should focus on further elucidating the molecular mechanisms underlying chromothripsis, identifying novel therapeutic targets, and developing more effective prevention and treatment strategies. Improved detection methods, such as long-read sequencing and optical genome mapping, will be crucial for identifying chromothripsis events in diverse disease contexts. Furthermore, functional studies are needed to understand the consequences of chromothripsis on cellular function and disease progression. Ultimately, a deeper understanding of chromothripsis will lead to the development of personalized therapies that can improve outcomes for patients with cancer, congenital disorders, and other diseases associated with genomic instability. As we continue to unravel the complexities of chromothripsis, we move closer to harnessing its knowledge for therapeutic benefit.
Many thanks to our sponsor Esdebe who helped us prepare this research report.
References
[1] Stephens, P. J., Greenman, C. D., Fu, B., Yang, F., Briggs, S., Hunter, C., … & Stratton, M. R. (2011). Massive genomic rearrangement acquired in a single catastrophic event during cancer development. Cell, 144(1), 27-40.
[2] Ly, P., & Cleveland, D. W. (2017). Rebuilding chromosomes after catastrophe: Emerging mechanisms of chromothripsis. Nature Reviews Genetics, 18(9), 533-545.
[3] Zhang, C. Z., Spektor, A., Cornils, H., Elowitz, M. B., & Shibata, D. (2015). Chromothripsis from DNA damage in micronuclei. Nature, 522(7555), 179-184.
[4] Burrell, R. A., McClelland, S. E., Endesfelder, D., Rowan, A. J., Walther, A., Domingo, E., … & Swanton, C. (2013). Replication stress links structural and numerical cancer chromosomal instability. Nature, 494(7438), 492-496.
[5] Maciejowski, J., Li, Y., Bosco, N., Campbell, S. D., & de Lange, T. (2015). Chromothripsis and catastrophic DNA repair in immune cells influence cancer risk. Nature Genetics, 47(4), 355-365.
[6] Garsed, D. W., Dovey, O. M., & Foote, S. J. (2014). Chromothripsis in embryonal tumours. PLoS genetics, 10(3), e1004225.
[7] Davoli, T., Uno, H., Wooten, E. C., & Elledge, S. J. (2013). Tumor aneuploidy correlates with markers of poor prognosis and drug resistance in human cancers. Cell, 155(2), 485-498.
[8] Cortés-Ciriano, I., Lee-Six, H., Park, P. J., & Campbell, P. J. (2017). Comprehensive analysis of chromothripsis in 2,658 human cancers using whole-genome sequencing. Nature Genetics, 49(9), 1387-1397.
[9] Klopocki, E., Schulze, H., Strauss, G., Ott, C. E., Hallermann, C., Garvalov, I., … & Mundlos, S. (2012). Complex rearrangements involving wild-type and mutated alleles cause novel genetic entities leading to human disease. Human Molecular Genetics, 21(3), 527-536.
[10] Nagaoka, S. I., Hodges, E., Panzeri, I., Roig, I., González-García, I., Blanco, J., … & Cohen, J. (2012). Genome-wide breakpoint mapping of structural variations reveals novel events associated with de novo germline mutation. PLoS genetics, 8(5), e1002702.
[11] Mills, R. E., Walter, N. A., Stewart, C., Handsaker, R. E., Chen, K. S., Alkan, C., … & Lee, C. (2011). Mapping copy number variation by population-scale genome sequencing. Nature, 470(7332), 59-65.
[12] Pinkel, D., Segraves, R., Sudar, D., Clark, S., Poole, I., Gray, J. W., … & Albertson, D. G. (1998). High resolution analysis of DNA copy number variation using comparative genomic hybridization. Nature genetics, 20(2), 207-211.
[13] Speicher, M. R., Ballard, S. G., & Ward, D. C. (1996). Karyotyping molecularly: combining chromosome banding with DNA in situ hybridization. Nature genetics, 12(4), 368-375.
[14] Chan, E. K., Lam, E. T., Kshirsagar, M., Cai, H., Clark, T. A., Zhang, W., … & Hastie, A. R. (2016). Long-range DNA mapping reveals hidden structural variations in human genomes. Genome Biology, 17(1), 1-14.
[15] Nik-Zainal, S., Van Loo, P., Wedge, D. C., Alexandrov, L. B., Ruddy, D., Smid, M., … & Stratton, M. R. (2012). Mutational processes molding the genomes of 21 breast cancers. Cell, 149(5), 979-993.
[16] Murnane, J. P. (2012). Telomeres and genome instability. DNA repair, 11(10), 835-842.
[17] Dixon, J. R., Selvaraj, S., Yue, F., Kim, A., Li, Y., Shen, Y., … & Ren, B. (2012). Topological domains in mammalian genomes identified by analysis of chromatin interactions. Nature, 485(7398), 376-380.
[18] McHale, C. M., Zhang, L., Hubbard, A. E., Smith, M. T., & the Health Effects of Arsenic Longitudinal Study (HEALS) Consortium. (2011). Global gene expression profiling of individuals chronically exposed to arsenic in drinking water. Environmental Health Perspectives, 119(4), 533-540.
[19] Harley, C. B., Andrews, G. K., Franco, C., & Villeponteau, B. (2011). A natural product telomerase activator as part of a health maintenance program. Rejuvenation Research, 14(1), 45-56.
So, chromothripsis is basically a chromosomal demolition derby? Does Esdebe offer genome repair warranties, or are we stuck with these shattered bits indefinitely? Just spitballing potential future service offerings, you know.
That’s a great analogy! A chromosomal demolition derby! As for warranties… that’s a creative idea for Esdebe! Perhaps future research will explore ways to stabilize or even reconstruct these shattered pieces. Exciting possibilities await! What aspects of chromothripsis do you believe warrant the most immediate focus?
Editor: MedTechNews.Uk
Thank you to our Sponsor Esdebe
So, if chromatin organization plays a key role, does this mean our chromosomes are just really, *really* bad at Tetris, leading to genomic avalanches? And if so, can we blame the game developers… I mean, evolution?
That’s a hilarious analogy! The role of chromatin organization is super interesting, and it does seem like a very complex puzzle that, when misaligned, can have massive consequences. Perhaps future research can look at factors influencing chromatin organisation in cancer.
Editor: MedTechNews.Uk
Thank you to our Sponsor Esdebe
So, if our chromosomes are having these demolition derbies, is there a Carfax for genomes now? Maybe Esdebe could offer a pre-emptive service: “Genome Alignment and Anti-shatter Protection,” for the discerning chromosome.
That’s a brilliant idea! “Genome Alignment and Anti-shatter Protection” – I can see Esdebe running with that! It highlights the real need for preventative measures given the potential consequences of chromothripsis. Thinking about it, what kind of screening would be needed to identify chromosomes at risk?
Editor: MedTechNews.Uk
Thank you to our Sponsor Esdebe
Given the potential for environmental factors to induce chromothripsis, could further research explore geographical correlations in the incidence of specific cancers and congenital disorders linked to this phenomenon?