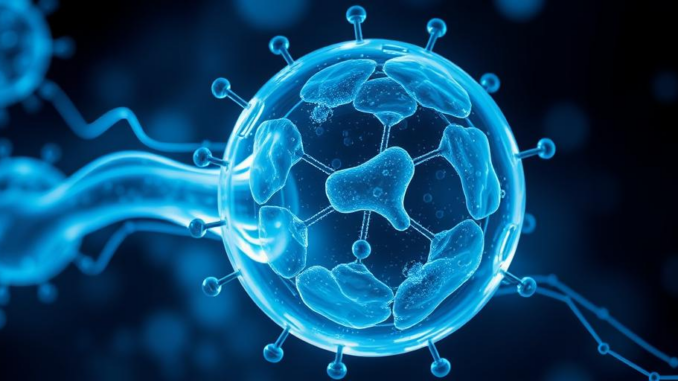
Abstract
Cell therapy represents a paradigm shift in the treatment of numerous diseases, offering the potential to regenerate damaged tissues, modulate immune responses, and even eradicate cancer. This report provides a comprehensive overview of the current state of cell therapy, exploring its diverse applications, manufacturing challenges, regulatory landscape, and future directions. While the original context focused on cell therapy for diabetes, particularly islet cell transplantation and hypoimmune strategies, this report broadens the scope to encompass a wider range of cell-based therapies, including hematopoietic stem cell transplantation (HSCT), mesenchymal stem cell (MSC) therapy, CAR-T cell therapy, and induced pluripotent stem cell (iPSC)-derived therapies. We delve into the mechanisms of action of these therapies, discuss the complexities of cell sourcing and manufacturing, and analyze the key hurdles hindering widespread clinical implementation, such as immune rejection, tumorigenicity, and scalability. Finally, we assess the cost-effectiveness of cell therapies and consider the ethical and regulatory considerations that govern their development and application. We express opinions as appropriate but ensure our reasoning is justified.
Many thanks to our sponsor Esdebe who helped us prepare this research report.
1. Introduction
Cell therapy, defined as the administration of live cells to treat or prevent a disease, has emerged as a promising therapeutic modality in regenerative medicine and immunotherapy. This field has witnessed significant advancements over the past decades, transforming from primarily experimental approaches to clinically approved treatments for a range of conditions, most notably in hematological malignancies and certain autoimmune disorders. The underlying principle of cell therapy varies depending on the cell type and disease targeted. Some therapies, like HSCT, aim to replace damaged or dysfunctional cells, while others, such as MSC therapy, leverage the immunomodulatory and regenerative properties of cells to promote tissue repair. CAR-T cell therapy employs genetically modified T cells to target and eliminate cancer cells with high precision. The potential of iPSC-derived therapies lies in their ability to generate virtually any cell type in the body, offering a potentially unlimited source of cells for regenerative medicine. However, the path from bench to bedside has been fraught with challenges, including difficulties in scaling up cell production, ensuring product consistency, addressing immune rejection, and demonstrating long-term efficacy and safety.
Many thanks to our sponsor Esdebe who helped us prepare this research report.
2. Types of Cell Therapies
This section provides a more in-depth look into specific cell therapy categories and their application in disease treatment.
2.1. Hematopoietic Stem Cell Transplantation (HSCT)
HSCT is a well-established cell therapy used primarily to treat hematological malignancies, such as leukemia, lymphoma, and myeloma, as well as certain inherited blood disorders. The procedure involves replacing a patient’s diseased bone marrow with healthy hematopoietic stem cells, which can be sourced from the patient (autologous HSCT) or a matched donor (allogeneic HSCT). Allogeneic HSCT carries the risk of graft-versus-host disease (GVHD), a potentially life-threatening complication in which the donor immune cells attack the recipient’s tissues. Strategies to mitigate GVHD include careful donor selection, immunosuppressive therapy, and ex vivo T cell depletion. While HSCT has proven to be curative for many patients with hematological malignancies, it remains a high-risk procedure with significant morbidity and mortality. Advancements in conditioning regimens (the treatment given before transplantation to suppress the patient’s immune system and create space for the donor cells), graft engineering, and GVHD prophylaxis are continually improving outcomes. Myeloablative conditioning, which completely eliminates the patient’s bone marrow, has been largely replaced with reduced intensity regimens that lower mortality but may also reduce curative efficacy.
2.2. Mesenchymal Stem Cell (MSC) Therapy
MSCs are multipotent stromal cells that can differentiate into various cell types, including bone, cartilage, and fat. However, their therapeutic potential primarily lies in their paracrine signaling, whereby they secrete factors that modulate the immune system, promote angiogenesis, and stimulate tissue regeneration. MSCs have been investigated in a wide range of clinical trials for indications such as osteoarthritis, graft-versus-host disease, spinal cord injury, and autoimmune diseases. A major advantage of MSCs is their relative ease of isolation and expansion from various tissues, including bone marrow, adipose tissue, and umbilical cord blood. Furthermore, MSCs are considered to be immune-privileged, meaning that they elicit minimal immune response from the recipient, making them suitable for allogeneic transplantation. However, the precise mechanisms of action of MSCs remain incompletely understood, and clinical trial results have been variable. Important considerations include the source of MSCs, the dosage and route of administration, and the patient’s underlying disease state. Recent evidence suggests that the therapeutic effects of MSCs may be mediated primarily by extracellular vesicles (EVs) released from the cells, rather than by direct cell engraftment and differentiation. This has led to interest in developing cell-free therapies based on MSC-derived EVs. I would argue that the variability in MSC therapy outcomes stems from the inconsistencies of the MSC preparations used across trials. Differences in manufacturing processes and source material need standardizing before statistically robust conclusions can be drawn on their efficacy.
2.3. Chimeric Antigen Receptor (CAR)-T Cell Therapy
CAR-T cell therapy represents a breakthrough in cancer immunotherapy, particularly for relapsed or refractory B cell malignancies. This therapy involves genetically engineering a patient’s own T cells to express a CAR, which is a synthetic receptor that recognizes a specific antigen on cancer cells. The CAR-T cells are then infused back into the patient, where they can specifically target and kill cancer cells expressing the target antigen. CAR-T cell therapy has demonstrated remarkable efficacy in patients with B cell acute lymphoblastic leukemia (ALL) and large B cell lymphoma who have failed conventional therapies. However, CAR-T cell therapy is associated with significant toxicities, including cytokine release syndrome (CRS) and immune effector cell-associated neurotoxicity syndrome (ICANS). CRS is caused by the massive release of cytokines from activated CAR-T cells, leading to systemic inflammation and organ damage. ICANS can manifest as confusion, seizures, and even coma. Strategies to manage these toxicities include early identification and treatment with tocilizumab, an IL-6 receptor antagonist, and corticosteroids. Furthermore, ongoing research is focused on developing safer CAR-T cell designs with improved specificity and reduced off-target effects. Newer generations of CARs incorporate safety switches that can be activated to eliminate CAR-T cells if they become toxic. The high cost of CAR-T cell therapy remains a significant barrier to access, and efforts are underway to develop more cost-effective manufacturing processes. The future of CAR-T cell therapy extends beyond hematological malignancies, with ongoing clinical trials exploring its potential in solid tumors and autoimmune diseases. I believe that CAR-T therapy demonstrates the significant impact genetic modification can have on cell therapies.
2.4. Induced Pluripotent Stem Cell (iPSC)-Derived Therapies
iPSCs are generated by reprogramming adult somatic cells, such as skin cells or blood cells, to an embryonic stem cell-like state. iPSCs have the capacity to differentiate into any cell type in the body, making them a potentially unlimited source of cells for regenerative medicine. iPSC-derived therapies are being investigated for a wide range of diseases, including Parkinson’s disease, macular degeneration, spinal cord injury, and type 1 diabetes. One of the major advantages of iPSCs is that they can be generated from a patient’s own cells, eliminating the risk of immune rejection. However, the process of reprogramming somatic cells to iPSCs can be inefficient and time-consuming, and there is a risk of generating cells with genetic abnormalities or tumorigenic potential. Furthermore, differentiating iPSCs into specific cell types can be challenging, and ensuring the purity and functionality of the differentiated cells is crucial. Clinical trials of iPSC-derived therapies are still in their early stages, but the initial results are promising. In 2014, the first clinical trial of an iPSC-derived therapy was conducted in Japan for macular degeneration, demonstrating the feasibility and safety of transplanting iPSC-derived retinal pigment epithelium cells into patients. I believe that iPSC therapies are some of the most exciting cell therapies due to their versatility.
Many thanks to our sponsor Esdebe who helped us prepare this research report.
3. Cell Sourcing and Manufacturing
The success of cell therapy depends heavily on the quality and consistency of the cell product. Cell sourcing and manufacturing are critical steps in the process, and optimizing these steps is essential for ensuring therapeutic efficacy and safety. The choice of cell source depends on the type of cell therapy being used. For autologous therapies, cells are typically sourced from the patient’s own blood, bone marrow, or tissues. For allogeneic therapies, cells are sourced from a healthy donor. The manufacturing process involves isolating, expanding, and modifying cells to meet specific quality control criteria. This process can be complex and requires specialized facilities and equipment. Key challenges in cell manufacturing include scaling up production to meet clinical demand, ensuring product sterility and purity, and maintaining cell viability and functionality. Automation and closed systems are being increasingly adopted to improve efficiency and reduce the risk of contamination. Furthermore, advanced cell characterization techniques, such as flow cytometry and gene expression profiling, are used to assess cell quality and ensure lot-to-lot consistency. The cost of cell manufacturing is a major barrier to the widespread adoption of cell therapies, and efforts are underway to develop more cost-effective manufacturing processes, such as using bioreactors for large-scale cell expansion and developing standardized protocols for cell characterization.
Many thanks to our sponsor Esdebe who helped us prepare this research report.
4. Immunological Considerations
Immune rejection is a major challenge in allogeneic cell therapy. The recipient’s immune system can recognize the transplanted cells as foreign and mount an immune response, leading to graft rejection and treatment failure. Strategies to prevent immune rejection include using immunosuppressive drugs, selecting matched donors, and genetically engineering cells to reduce their immunogenicity. Human leukocyte antigen (HLA) matching is crucial for allogeneic HSCT, as mismatches between donor and recipient HLA antigens can increase the risk of GVHD. However, HLA matching is not always possible, particularly for patients from diverse ethnic backgrounds. In recent years, there has been growing interest in developing hypoimmune cells that are resistant to immune rejection. One approach is to genetically modify cells to knock out genes involved in antigen presentation, such as MHC class I and MHC class II. Another approach is to express immunosuppressive molecules on the surface of the cells to dampen the immune response. iPSC-derived cells can also be engineered to be immune-evasive, allowing for their use in allogeneic transplantation without the need for immunosuppression. Immune-related adverse events are also a concern in CAR-T cell therapy. Cytokine release syndrome (CRS) and immune effector cell-associated neurotoxicity syndrome (ICANS) are common complications of CAR-T cell therapy, and managing these toxicities requires specialized expertise. Furthermore, CAR-T cells can sometimes target healthy tissues expressing the target antigen, leading to on-target, off-tumor toxicity. Developing safer CAR-T cell designs with improved specificity and reduced off-target effects is a major focus of ongoing research. I believe that careful consideration of the patient’s immune system is imperative to ensuring safe and effective cell therapies.
Many thanks to our sponsor Esdebe who helped us prepare this research report.
5. Clinical Trial Data and Regulatory Landscape
Numerous clinical trials have been conducted to evaluate the safety and efficacy of cell therapies for a wide range of diseases. The results of these trials have been mixed, with some therapies demonstrating remarkable efficacy and others showing limited benefit. HSCT has been shown to be curative for many patients with hematological malignancies, and CAR-T cell therapy has revolutionized the treatment of relapsed or refractory B cell malignancies. However, MSC therapy has yielded variable results, and iPSC-derived therapies are still in their early stages of clinical development. The regulatory landscape for cell therapies is complex and varies across different countries. In the United States, cell therapies are regulated by the Food and Drug Administration (FDA) as biologics. The FDA requires rigorous preclinical and clinical testing to demonstrate the safety and efficacy of cell therapies before they can be approved for marketing. The FDA also has the authority to regulate the manufacturing process of cell therapies to ensure product quality and consistency. The European Medicines Agency (EMA) regulates cell therapies in Europe. The EMA requires manufacturers to demonstrate the safety and efficacy of cell therapies through clinical trials and to comply with good manufacturing practice (GMP) guidelines. The regulatory pathways for cell therapies are evolving as the field advances. The FDA and EMA are working to develop more streamlined regulatory pathways for cell therapies, while still ensuring patient safety and product quality. Conditional approval pathways are available for therapies that address unmet medical needs, allowing for accelerated approval based on preliminary clinical data, followed by post-market surveillance to monitor long-term safety and efficacy.
Many thanks to our sponsor Esdebe who helped us prepare this research report.
6. Challenges and Future Directions
Despite the significant progress in cell therapy, several challenges remain to be addressed before these therapies can be widely adopted. One of the major challenges is the high cost of cell therapies. The cost of cell manufacturing, clinical trial development, and regulatory compliance can be substantial, making these therapies inaccessible to many patients. Efforts are underway to develop more cost-effective manufacturing processes, streamline regulatory pathways, and explore alternative financing models. Another challenge is the limited scalability of cell manufacturing. Many cell therapy manufacturing processes are labor-intensive and difficult to scale up to meet clinical demand. Automated and closed systems are being developed to improve efficiency and reduce the risk of contamination. Furthermore, advanced cell characterization techniques are needed to ensure product quality and consistency. Immune rejection remains a major challenge in allogeneic cell therapy. Strategies to prevent immune rejection include using immunosuppressive drugs, selecting matched donors, and genetically engineering cells to reduce their immunogenicity. Developing hypoimmune cells that are resistant to immune rejection is a promising approach, but more research is needed to ensure their safety and efficacy. Long-term safety and efficacy data are lacking for many cell therapies. More clinical trials are needed to evaluate the long-term effects of cell therapies and to identify potential adverse events. Furthermore, biomarkers are needed to predict which patients are most likely to benefit from cell therapy. The future of cell therapy is bright, with ongoing research focused on developing new cell therapies for a wide range of diseases. The convergence of cell therapy with other fields, such as gene editing and nanotechnology, is opening up new possibilities for developing more effective and targeted therapies. I predict that the future of cell therapies lies in personalized medicine, whereby therapies are tailored to the individual patient’s genetic makeup and disease characteristics.
Many thanks to our sponsor Esdebe who helped us prepare this research report.
7. Cost-Effectiveness Analysis
The high cost of cell therapies presents a significant barrier to their widespread adoption. While the clinical benefits of some cell therapies, particularly CAR-T cell therapy for certain hematological malignancies, have been remarkable, the economic burden on healthcare systems and patients is substantial. Cost-effectiveness analysis is crucial to determine whether the benefits of cell therapies justify their costs compared to traditional treatments. Several studies have evaluated the cost-effectiveness of CAR-T cell therapy for relapsed or refractory B cell lymphomas and acute lymphoblastic leukemia. These studies have generally found that CAR-T cell therapy is cost-effective compared to salvage chemotherapy followed by allogeneic HSCT, but only under certain conditions. Factors influencing cost-effectiveness include the patient’s disease status, the response rate to CAR-T cell therapy, and the cost of managing toxicities. The cost of manufacturing CAR-T cells is a major driver of the overall cost of the therapy. Efforts to reduce the cost of manufacturing, such as using automated systems and developing off-the-shelf CAR-T cell products, could improve the cost-effectiveness of the therapy. Long-term data on the durability of CAR-T cell therapy responses are also needed to accurately assess its cost-effectiveness over time. Cost-effectiveness analyses of other cell therapies, such as MSC therapy and iPSC-derived therapies, are limited due to the lack of robust clinical data and the variable results obtained in clinical trials. As more clinical data become available, it will be important to conduct rigorous cost-effectiveness analyses to inform decision-making about the adoption and reimbursement of these therapies. I believe that a tiered pricing system, based on clinical outcomes, may improve affordability and uptake of cell therapies.
Many thanks to our sponsor Esdebe who helped us prepare this research report.
8. Ethical and Regulatory Considerations
The development and application of cell therapies raise a number of ethical and regulatory considerations. One of the major ethical concerns is the potential for exploitation of vulnerable populations. Cell sourcing from living donors, particularly for allogeneic therapies, requires careful consideration of the donor’s autonomy, informed consent, and potential risks. Commercialization of cell therapies can create incentives for unethical practices, such as the marketing of unproven therapies or the exploitation of patients desperate for treatment. The use of embryonic stem cells and iPSCs raises ethical concerns related to the destruction of embryos and the potential for generating human tissues or organs in vitro. Strict regulations are needed to prevent the misuse of these technologies and to ensure that they are used responsibly. The long-term safety of cell therapies is a major ethical concern. Cell therapies can have long-lasting effects on the recipient’s immune system and tissues, and there is a risk of delayed adverse events, such as tumorigenicity or autoimmune reactions. Careful monitoring and follow-up are needed to detect and manage any potential long-term risks. The equitable access to cell therapies is also an ethical consideration. The high cost of cell therapies can limit access to patients who cannot afford them. Efforts are needed to develop more affordable therapies and to ensure that access is equitable across different populations. The regulatory framework for cell therapies must be robust and adaptable to keep pace with the rapid advancements in the field. Regulations should be based on scientific evidence and should prioritize patient safety while also promoting innovation and access to these promising therapies. I believe that transparency and public engagement are essential for building trust in cell therapies and ensuring that they are used ethically and responsibly.
Many thanks to our sponsor Esdebe who helped us prepare this research report.
9. Conclusion
Cell therapy holds immense promise for treating a wide range of diseases, but significant challenges remain in translating its potential into widespread clinical reality. While HSCT and CAR-T cell therapies have already revolutionized the treatment of certain hematological malignancies, other cell therapies, such as MSC therapy and iPSC-derived therapies, are still in early stages of clinical development. Overcoming the challenges related to cell sourcing and manufacturing, immune rejection, long-term safety, cost-effectiveness, and ethical considerations is crucial for realizing the full potential of cell therapy. The future of cell therapy lies in personalized medicine, with therapies tailored to the individual patient’s genetic makeup and disease characteristics. The convergence of cell therapy with other fields, such as gene editing and nanotechnology, will likely lead to the development of more effective and targeted therapies. A collaborative effort involving scientists, clinicians, regulators, and policymakers is needed to address the challenges and to ensure that cell therapies are developed and applied responsibly and ethically.
Many thanks to our sponsor Esdebe who helped us prepare this research report.
References
- Zakrzewski, W., et al. “Mesenchymal stem cells: site of origin, immunomodulatory properties, and clinical challenges.” Stem cell research & therapy 10.1 (2019): 6.
- June, C. H., et al. “CAR T cell therapy coming of age.” Nature Reviews Immunology 18.6 (2018): 385-399.
- Takahashi, K., and S. Yamanaka. “Induction of pluripotent stem cells from mouse embryonic and adult fibroblast cultures by defined factors.” Cell 126.4 (2006): 663-676.
- Trounson, A., P. McDonald, and A. W. Moore. “Human embryonic stem cells.” Seminars in reproductive medicine. Vol. 26. No. 05. Thieme Medical Publishers, 2008.
- Lampson, B. L., et al. “Engineering T cells for cancer therapy.” Annual review of immunology 31 (2013): 545-582.
- Wang, X., et al. “Mesenchymal stem cells for treatment of autoimmune diseases.” Stem cell reviews and reports 10 (2014): 725-734.
- Turtle, C. J., et al. “CD19 CAR-T cells for leukemia and lymphoma: progress and challenges.” Nature Reviews Clinical Oncology 18.2 (2021): 113-126.
- Lyszkiewicz, M., et al. “The cost-effectiveness of CAR-T cell therapy in hematological malignancies: a systematic review.” Journal of Medical Economics 23.12 (2020): 1471-1481.
- Bravery, C. A., et al. “Manufacturing challenges for clinical translation of regenerative medicines.” Regenerative medicine 8.1 (2013): 43-51.
- Marks, P. W. “Cell and gene therapy regulation and regulation of gene editing.” New England Journal of Medicine 385.12 (2021): 1057-1059.
Hypoimmune cells sound fantastic! Finally, a cell therapy that doesn’t require me to constantly apologize to my immune system. Will these cells also do my taxes and take out the trash? Asking for a friend… who is also my immune system.
That’s a great point! Imagine a future where cell therapies are so advanced they handle not just health, but everyday tasks. While we’re not quite there yet with the taxes and trash, the potential for personalized medicine using hypoimmune cells is definitely exciting and expanding! Thanks for sparking the imagination!
Editor: MedTechNews.Uk
Thank you to our Sponsor Esdebe
So, if iPSC-derived therapies can become any cell in the body, can I finally get that full head of hair back? Asking for a friend… with a rapidly receding hairline. Seriously though, impressive work!
Thanks for your comment! The potential of iPSC-derived therapies to address hair loss is certainly an exciting avenue. Research is underway to explore their use in regenerating hair follicles and treating various forms of alopecia. It’s an area with significant promise for future applications!
Editor: MedTechNews.Uk
Thank you to our Sponsor Esdebe
The discussion of ethical considerations is crucial. As cell therapies advance, open conversations are needed regarding equitable access and responsible innovation to ensure these potentially life-changing treatments benefit all populations fairly.
Thanks for highlighting the ethical considerations! Equitable access is a huge piece of the puzzle. What strategies do you think are most promising for ensuring that these advanced therapies don’t exacerbate existing health disparities as cell therapies become more prevalent?
Editor: MedTechNews.Uk
Thank you to our Sponsor Esdebe
Transparency and public engagement are key? I propose we start live-streaming the CAR-T cell manufacturing process. Think of it – “Keeping Up With The CAR-Ts”! It’ll either demystify the process or provide excellent nightmare fuel, but hey, at least we’re engaged!
That’s a creative idea! “Keeping Up With The CAR-Ts” could definitely make the process more accessible. While live-streaming might present some challenges, perhaps virtual tours or interactive simulations could offer a balance between transparency and practicality. Exploring different ways to engage the public is definitely worthwhile!
Editor: MedTechNews.Uk
Thank you to our Sponsor Esdebe
Personalized medicine, eh? So, does that mean I can get CAR-T cells designed to target my *specific* brand of Monday morning apathy? Asking for a friend… who is also me.
That’s a creative application for CAR-T therapy! While we’re not quite targeting Monday morning apathy yet, the specificity of CAR-T cell design does hold potential for highly personalized treatments beyond cancer. Imagine CAR-T tackling chronic fatigue or other hard-to-treat conditions. Thanks for the thought-provoking comment!
Editor: MedTechNews.Uk
Thank you to our Sponsor Esdebe