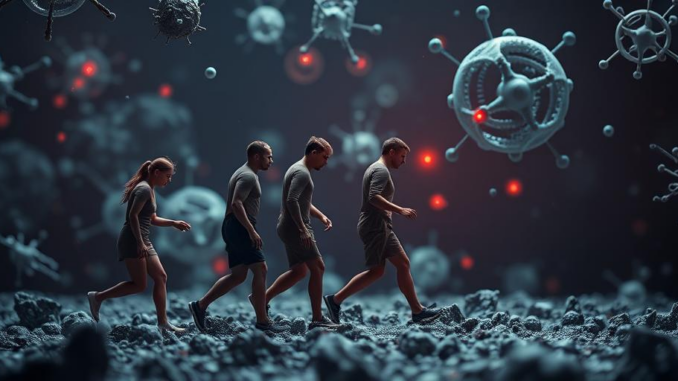
Abstract
Mutations, often described as alterations in the genomic sequence, are fundamental drivers of both evolution and disease. While frequently perceived negatively due to their association with genetic disorders and cancer, mutations also underpin the adaptability and diversity of life. This report delves into the intricate landscape of mutations, exploring their diverse types, underlying causes, and multifaceted consequences at the molecular, cellular, and organismal levels. We examine the mechanisms by which mutations impact protein function and contribute to disease pathogenesis, focusing on key examples across various human pathologies. Furthermore, we investigate the cutting-edge technologies employed for mutation detection and analysis, highlighting their pivotal role in advancing personalized medicine and the development of targeted therapies. Finally, we broaden the scope to consider the evolutionary implications of mutations, underscoring their indispensable role in shaping the genetic variation and adaptation of species over time. We conclude by discussing the ethical considerations arising from our increasing ability to manipulate and correct mutations, emphasizing the need for responsible innovation in this rapidly evolving field.
Many thanks to our sponsor Esdebe who helped us prepare this research report.
1. Introduction
The concept of mutation, initially introduced by Hugo de Vries at the dawn of the 20th century, has undergone a profound transformation. From its early association with discrete and readily observable phenotypic changes, mutation is now understood as a complex and multifaceted process encompassing alterations ranging from single nucleotide substitutions to large-scale chromosomal rearrangements. While mutations are frequently linked to disease, it is crucial to recognize that they are also the raw material for evolution, providing the genetic variation upon which natural selection acts. Therefore, a balanced perspective is essential, acknowledging both the detrimental and beneficial consequences of mutations.
The study of mutations has been revolutionized by advancements in DNA sequencing technologies. The ability to rapidly and accurately determine the nucleotide sequence of entire genomes has enabled researchers to identify and characterize mutations with unprecedented resolution. This has led to a deeper understanding of the mutational landscape of various organisms, including humans, and has facilitated the development of novel diagnostic and therapeutic strategies.
This report provides a comprehensive overview of the diverse aspects of mutations, encompassing their molecular mechanisms, functional consequences, and evolutionary significance. We aim to provide an expert-level perspective on this critical topic, highlighting recent advances and future directions in the field.
Many thanks to our sponsor Esdebe who helped us prepare this research report.
2. Types and Mechanisms of Mutations
Mutations can be classified based on various criteria, including the scale of the alteration, the type of nucleotide change, and the mechanism by which the mutation arises.
2.1 Classification by Scale
- Point Mutations: These involve alterations at a single nucleotide position. They can be further subdivided into:
- Substitutions: Replacement of one nucleotide with another. These can be transitions (purine to purine or pyrimidine to pyrimidine) or transversions (purine to pyrimidine or vice versa).
- Insertions: Addition of one or more nucleotides into the DNA sequence.
- Deletions: Removal of one or more nucleotides from the DNA sequence.
- Small Insertions and Deletions (Indels): These involve the insertion or deletion of a small number of nucleotides, typically less than 50 base pairs.
- Large-Scale Mutations: These encompass larger alterations in the genome, including:
- Chromosomal Rearrangements: Changes in the structure or number of chromosomes, such as deletions, duplications, inversions, and translocations.
- Copy Number Variations (CNVs): Alterations in the number of copies of a particular DNA segment.
- Aneuploidy: Abnormal number of chromosomes, such as trisomy (three copies of a chromosome) or monosomy (one copy of a chromosome).
2.2 Mechanisms of Mutation
Mutations can arise through a variety of mechanisms, broadly classified as spontaneous or induced.
- Spontaneous Mutations: These occur due to inherent errors in cellular processes, such as DNA replication and repair. Examples include:
- DNA Replication Errors: DNA polymerases, while generally highly accurate, can occasionally incorporate incorrect nucleotides. Mismatched base pairs can lead to mutations if not corrected by proofreading or mismatch repair mechanisms.
- Spontaneous Chemical Modifications: DNA bases can undergo spontaneous chemical modifications, such as deamination of cytosine to uracil. If unrepaired, uracil will pair with adenine during replication, leading to a C to T transition.
- Replication Slippage: During replication, the DNA polymerase can slip on repetitive sequences, leading to the insertion or deletion of nucleotides. This is particularly common in microsatellite regions.
- Induced Mutations: These are caused by external agents, known as mutagens. Mutagens can be physical (e.g., radiation) or chemical (e.g., alkylating agents).
- Radiation: Ionizing radiation (e.g., X-rays, gamma rays) can cause DNA strand breaks and base damage. Ultraviolet (UV) radiation can cause the formation of pyrimidine dimers, which distort the DNA structure and interfere with replication.
- Chemical Mutagens: These include a diverse range of compounds that can directly modify DNA bases, intercalate between base pairs, or interfere with DNA replication or repair. Examples include alkylating agents, intercalating agents, and base analogs.
It’s important to consider the context-dependent nature of mutation rates. Some genomic regions are inherently more susceptible to mutation than others, due to factors such as local DNA sequence, chromatin structure, and accessibility to repair mechanisms. Furthermore, the activity of DNA repair pathways can vary between different cell types and tissues, influencing the overall mutation rate.
Many thanks to our sponsor Esdebe who helped us prepare this research report.
3. Effects of Mutations on Protein Function and Disease Development
The consequences of mutations on protein function can vary widely, ranging from negligible effects to complete loss of function. The impact of a mutation depends on several factors, including the location of the mutation within the gene, the nature of the amino acid change (if any), and the role of the affected protein in cellular processes.
3.1 Missense Mutations
Missense mutations result in the substitution of one amino acid for another in the protein sequence. The effect of a missense mutation depends on the chemical properties of the substituted amino acid and its location within the protein. Some missense mutations may have little or no effect on protein function if the substituted amino acid is similar in size and charge to the original amino acid, or if the mutation occurs in a non-critical region of the protein. However, other missense mutations can disrupt protein folding, stability, or activity, leading to impaired function.
3.2 Nonsense Mutations
Nonsense mutations introduce a premature stop codon into the mRNA sequence, resulting in a truncated protein. The severity of the effect depends on the location of the stop codon. If the stop codon is located early in the coding sequence, the resulting protein may be completely non-functional and rapidly degraded. Nonsense mutations can often trigger nonsense-mediated decay (NMD), a cellular mechanism that degrades mRNAs containing premature stop codons, further reducing the amount of the truncated protein.
3.3 Frameshift Mutations
Frameshift mutations result from the insertion or deletion of a number of nucleotides that is not a multiple of three. This shifts the reading frame of the mRNA, leading to the incorporation of incorrect amino acids downstream of the mutation. Frameshift mutations typically result in a completely non-functional protein or a protein with altered properties.
3.4 Splice Site Mutations
Splice site mutations affect the splicing of pre-mRNA, leading to aberrant mRNA transcripts. These mutations can result in exon skipping, intron retention, or the use of cryptic splice sites. The consequences of splice site mutations can be diverse, depending on the specific splicing defect and the function of the affected exon(s).
3.5 Mutations in Non-Coding Regions
While mutations within protein-coding regions are often the focus of attention, mutations in non-coding regions can also have significant effects on gene expression and cellular function. For example, mutations in promoter regions can alter the binding affinity of transcription factors, leading to changes in gene transcription. Mutations in enhancers or silencers can affect the activity of these regulatory elements, influencing gene expression in specific tissues or developmental stages. Mutations in microRNA (miRNA) genes or their binding sites can alter the regulation of gene expression by miRNAs. Mutations in long non-coding RNAs (lncRNAs) can disrupt their interactions with other molecules, affecting various cellular processes. Mutations in telomeres can affect cellular senescence and genome stability.
3.6 Mutations and Disease
Mutations play a critical role in the development of a wide range of diseases, including cancer, genetic disorders, and infectious diseases. Specific examples include:
- Cancer: Cancer is fundamentally a genetic disease, driven by the accumulation of mutations in genes that regulate cell growth, division, and differentiation. Mutations in oncogenes (genes that promote cell growth) and tumor suppressor genes (genes that inhibit cell growth) are particularly important in cancer development. Examples include mutations in TP53 (a tumor suppressor gene), KRAS (an oncogene), and EGFR (an oncogene).
- Genetic Disorders: Many genetic disorders are caused by mutations in single genes. Examples include cystic fibrosis (caused by mutations in the CFTR gene), sickle cell anemia (caused by a mutation in the HBB gene), and Huntington’s disease (caused by a mutation in the HTT gene).
- Infectious Diseases: Mutations in pathogens, such as viruses and bacteria, can lead to drug resistance and increased virulence. For example, mutations in the HIV reverse transcriptase gene can confer resistance to antiviral drugs. Mutations in bacteria can lead to antibiotic resistance.
Many thanks to our sponsor Esdebe who helped us prepare this research report.
4. Mutation Detection and Analysis Technologies
A variety of technologies are available for detecting and analyzing mutations, each with its own strengths and limitations.
4.1 DNA Sequencing
DNA sequencing is the gold standard for mutation detection. Several sequencing technologies are available, including:
- Sanger Sequencing: This is a first-generation sequencing method that is highly accurate but relatively slow and expensive. It is typically used for sequencing individual genes or small genomic regions.
- Next-Generation Sequencing (NGS): NGS technologies enable the rapid and cost-effective sequencing of entire genomes or exomes (the protein-coding regions of the genome). NGS is widely used for mutation discovery and diagnostics. Various NGS platforms are available, including Illumina, Ion Torrent, and PacBio.
- Third-Generation Sequencing: These technologies, such as PacBio and Oxford Nanopore, can read much longer DNA fragments than NGS technologies. This is useful for resolving complex genomic regions and detecting structural variations.
4.2 Polymerase Chain Reaction (PCR)-Based Methods
PCR-based methods are used to amplify specific DNA regions, enabling the detection of mutations in these regions. Examples include:
- Allele-Specific PCR (AS-PCR): This method uses primers that are specific for a particular allele, allowing for the detection of known mutations.
- High-Resolution Melting (HRM) Analysis: This method detects mutations based on differences in the melting temperature of DNA fragments.
- Quantitative PCR (qPCR): This method can be used to quantify the amount of a particular DNA sequence, allowing for the detection of copy number variations.
4.3 Microarrays
Microarrays are used to detect mutations by hybridizing DNA samples to probes that are specific for different DNA sequences. Microarrays can be used to detect single nucleotide polymorphisms (SNPs), copy number variations, and other types of mutations.
4.4 Digital PCR (dPCR)
dPCR is a highly sensitive method that allows for the absolute quantification of DNA molecules. dPCR can be used to detect rare mutations in a background of normal DNA, making it useful for detecting circulating tumor DNA (ctDNA) in cancer patients.
4.5 CRISPR-Based Detection
The CRISPR-Cas system, known for its genome editing capabilities, is also being adapted for mutation detection. Methods like SHERLOCK and DETECTR utilize Cas enzymes to specifically cleave target DNA or RNA sequences, allowing for highly sensitive and specific detection of mutations. These techniques are particularly promising for point-of-care diagnostics and high-throughput screening.
The selection of the appropriate mutation detection technology depends on the specific research question or clinical application. Factors to consider include the type of mutation being investigated, the required sensitivity and specificity, the cost and throughput of the technology, and the availability of expertise and resources.
Many thanks to our sponsor Esdebe who helped us prepare this research report.
5. Mutations in Personalized Medicine and Targeted Therapies
The identification and characterization of mutations have revolutionized the field of medicine, leading to the development of personalized medicine approaches and targeted therapies. Personalized medicine involves tailoring medical treatment to the individual characteristics of each patient, including their genetic makeup. Targeted therapies are drugs that specifically target cancer cells that have specific mutations.
5.1 Personalized Cancer Therapy
The genomic landscape of cancer varies greatly between individuals and even within the same tumor. Identifying specific mutations in cancer cells can guide the selection of targeted therapies that are most likely to be effective. For example, patients with lung cancer who have mutations in the EGFR gene can be treated with EGFR inhibitors. Patients with melanoma who have mutations in the BRAF gene can be treated with BRAF inhibitors. The use of targeted therapies has significantly improved the outcomes for many cancer patients.
5.2 Genetic Screening and Counseling
Genetic screening can identify individuals who are at increased risk of developing certain diseases due to inherited mutations. This information can be used to provide genetic counseling and to implement preventative measures, such as lifestyle changes or prophylactic surgery. For example, women who have mutations in the BRCA1 or BRCA2 genes are at increased risk of developing breast and ovarian cancer. These women may choose to undergo prophylactic mastectomies or oophorectomies to reduce their risk.
5.3 Gene Therapy
Gene therapy involves the introduction of genetic material into cells to treat or prevent disease. Gene therapy can be used to correct mutations that cause genetic disorders or to introduce new genes that provide a therapeutic benefit. Several gene therapy products have been approved for the treatment of genetic disorders, such as spinal muscular atrophy and inherited blindness.
The ethical implications of using gene therapy for germline editing (modifying the DNA of sperm or eggs) are complex and controversial. Germline editing could potentially correct genetic defects and prevent the transmission of disease to future generations. However, it also raises concerns about unintended consequences, off-target effects, and the potential for eugenics. Careful consideration and regulation are needed to ensure the responsible development and use of gene therapy technologies.
Many thanks to our sponsor Esdebe who helped us prepare this research report.
6. Evolutionary Significance of Mutations
Mutations are the raw material for evolution, providing the genetic variation upon which natural selection acts. Without mutations, there would be no new traits for natural selection to favor, and evolution would grind to a halt. Most mutations are either neutral or deleterious, but a small fraction of mutations are beneficial, providing a selective advantage to the organism. Over time, beneficial mutations can accumulate in populations, leading to adaptation and the evolution of new species.
6.1 Adaptation
Mutations allow organisms to adapt to changing environments. For example, mutations that confer resistance to antibiotics have allowed bacteria to survive in the presence of antibiotics. Mutations that allow plants to tolerate drought have allowed them to colonize arid environments. The ability to adapt to changing environments is essential for the survival of species.
6.2 Speciation
Mutations can contribute to the formation of new species. When populations become reproductively isolated, mutations can accumulate independently in each population. Over time, these mutations can lead to genetic divergence, eventually resulting in the formation of new species. The process of speciation is driven by mutation, genetic drift, and natural selection.
6.3 Mutation Rate Evolution
Intriguingly, mutation rates themselves are subject to evolutionary pressures. While high mutation rates can lead to a greater supply of novel genetic variants, they also increase the risk of deleterious mutations. Therefore, organisms must strike a balance between the benefits of increased genetic variation and the costs of increased mutation load. The optimal mutation rate may depend on factors such as population size, environmental stability, and the frequency of adaptive opportunities.
Many thanks to our sponsor Esdebe who helped us prepare this research report.
7. Future Directions and Ethical Considerations
Research into mutations is a rapidly evolving field with numerous promising avenues for future exploration. Some key areas of focus include:
- Developing more accurate and efficient mutation detection technologies: This includes improving the sensitivity and specificity of existing technologies, as well as developing new technologies that can detect mutations in a high-throughput manner.
- Understanding the factors that influence mutation rates: This includes studying the roles of DNA repair pathways, chromatin structure, and environmental factors in determining mutation rates.
- Developing new therapeutic strategies for targeting mutations: This includes developing drugs that can specifically target cancer cells with specific mutations, as well as developing gene therapy approaches to correct mutations that cause genetic disorders.
- Exploring the role of mutations in aging and other complex diseases: This includes studying the accumulation of mutations over time and their contribution to age-related diseases.
As our ability to manipulate and correct mutations increases, it is essential to consider the ethical implications of these technologies. Key ethical considerations include:
- The safety and efficacy of gene therapy: Gene therapy has the potential to correct genetic defects, but it also carries risks of off-target effects and unintended consequences. Careful testing and regulation are needed to ensure the safety and efficacy of gene therapy products.
- The ethical implications of germline editing: Germline editing could potentially correct genetic defects and prevent the transmission of disease to future generations. However, it also raises concerns about unintended consequences, off-target effects, and the potential for eugenics. Careful consideration and regulation are needed to ensure the responsible development and use of germline editing technologies.
- The accessibility and affordability of personalized medicine: Personalized medicine has the potential to improve the outcomes for many patients, but it is also expensive and may not be accessible to all. Efforts are needed to ensure that personalized medicine is available to all patients who could benefit from it.
Many thanks to our sponsor Esdebe who helped us prepare this research report.
8. Conclusion
Mutations are a fundamental aspect of biology, playing a critical role in both evolution and disease. Advances in DNA sequencing and other technologies have enabled researchers to study mutations with unprecedented resolution, leading to a deeper understanding of their molecular mechanisms, functional consequences, and evolutionary significance. The identification and characterization of mutations have revolutionized the field of medicine, leading to the development of personalized medicine approaches and targeted therapies. As our ability to manipulate and correct mutations increases, it is essential to consider the ethical implications of these technologies and to ensure that they are used responsibly to benefit humanity.
Many thanks to our sponsor Esdebe who helped us prepare this research report.
References
- Alberts, B., Johnson, A., Lewis, J., Raff, M., Roberts, K., & Walter, P. (2002). Molecular biology of the cell. New York: Garland Science.
- Campbell, I. H., & Tomlinson, I. P. M. (2013). The cancer genome. Cold Spring Harbor Perspectives in Medicine, 3(11), a010219.
- Drake, J. W. (1991). A constant rate of spontaneous mutation in DNA-based microbes. Proceedings of the National Academy of Sciences, 88(16), 7160-7164.
- Frankham, R., Ballou, J. D., & Briscoe, D. A. (2002). Introduction to conservation genetics. Cambridge University Press.
- Griffiths, A. J. F., Wessler, S. R., Carroll, S. B., & Doebley, J. (2015). Introduction to genetic analysis. Macmillan.
- Li, H. (2014). Aligning sequence reads, clone sequences and assembly contigs with BWA-MEM. arXiv preprint arXiv:1303.3997.
- Lodish, H., Berk, A., Zipursky, S. L., Matsudaira, P., Baltimore, D., & Darnell, J. (2000). Molecular cell biology. New York: W. H. Freeman.
- McFarland, C. D., Mirny, L. A., & Desai, M. M. (2013). The dual role of mutation in bacterial adaptation. Proceedings of the National Academy of Sciences, 110(10), 3747-3752.
- Pelizzola, M., & Ecker, J. R. (2021). Genome-wide technologies for mapping DNA methylation. Nature Reviews Genetics, 22(8), 493-509.
- Vogelstein, B., Papadopoulos, N., Velculescu, V. E., Zhou, S., Diaz Jr, L. A., Kinzler, K. W. (2013). Cancer genome landscapes. Science, 339(6127), 1546-1558.
So, mutations are like typos in the book of life? Some are no big deal, others change the whole plot, and occasionally, one makes the story way more interesting! Glad we have Esdebe proofreading, or we’d all be stuck in the Stone Age.
That’s a great analogy! The “typo” mutations driving evolution are fascinating. It makes you wonder how many beneficial “typos” are still waiting to be discovered, or how many seemingly bad ones have latent potential. Thanks for the comment!
Editor: MedTechNews.Uk
Thank you to our Sponsor Esdebe
So mutations are nature’s way of saying, “Let’s see what happens if we swap this bit for that?” Like accidentally ordering pineapple on pizza…sometimes it’s surprisingly good, often it’s a learning experience, but always memorable!